Chapter 8: High-Efficacy Stimulants
2nd edition as of August 2022; Chapter updated Jan 2024
Chapter Overview
Now that we have covered the fundamentals in Unit 1, it is time for us to start examining specific types of drugs. For the remainder of this book, each chapter will introduce a new class of psychoactive drugs and focus on a few key examples. Be prepared to apply the concepts covered in the first unit when learning about each drug. In this unit, we will examine stimulants and depressants, starting with high-efficacy stimulants.
Chapter Outline
- 8.1.1. Stimulant Overview
- 8.1.2. High-Efficacy vs. Low-Efficacy Stimulants
- 8.2.1. Drug History and Overview
- 8.2.2. Administration and Pharmacokinetics
- 8.2.3. Mechanisms of Action and Effects
- 8.3.1. Drug History and Overview
- 8.3.2. Administration and Pharmacokinetics
- 8.3.3. Mechanisms of Action and Effects
Chapter Learning Outcomes
- Distinguish high- and low-efficacy stimulants.
- Describe the history, administration, mechanisms of action, and effects of cocaine.
- Describe the history, administration, mechanisms of action, and effects of amphetamines.
8.1. CNS Stimulants
Section Learning Objectives
- Explain how sympathomimetic drugs enhance CNS activity.
- Differentiate between high-efficacy and low-efficacy stimulants.
The first class of drugs we will be looking at are stimulants. A stimulant is any drug that increases the activity of the central nervous system. For this reason, they are also known as CNS stimulants or “uppers” due to their stimulating effects. Before examining any specific drugs, we will first go over some properties that are common across all stimulants.
8.1.1. Stimulant Overview
As mentioned, stimulants tend to increase CNS activity. A common method for doing so involves enhancing the effects of the sympathetic nervous system. Recall that this is the division of the autonomic nervous system that is responsible for our “fight-or-flight” response. When our sympathetic nervous system is activated, we enter a state of heightened arousal. Our bodies prepare for action by increasing our heart rate, dilating pupils, constricting blood vessels, increasing blood flow to the skeletal muscles, and causing various other physiological changes.
Stimulants target the pathways of the catecholamines norepinephrine and dopamine in the CNS and the sympathetic nervous system. Many stimulants, including the ones in this chapter, possess direct or indirect agonist activity at adrenergic receptors. These drugs are called sympathomimetic drugs because they evoke physiological changes that mimic the activation of the sympathetic nervous system.
Most stimulants cause euphoria and agitation while increasing energy, alertness, confidence, and sexual desire. Many stimulants have therapeutic uses, although the pleasurable effects and addictive properties of the drugs mean that they are also used frequently for recreational purposes.
8.1.2. High-Efficacy vs. Low-Efficacy Stimulants
Not all stimulants produce the same level of effect. Think back to the dose-response curves that you studied in Chapter 7 and how the height of the curve indicated efficacy or the maximum possible effect of the drug. Some drugs have lower ceiling effects than others. This is the case for stimulants, which we can group into two main categories—high-efficacy stimulants and low-efficacy ones.
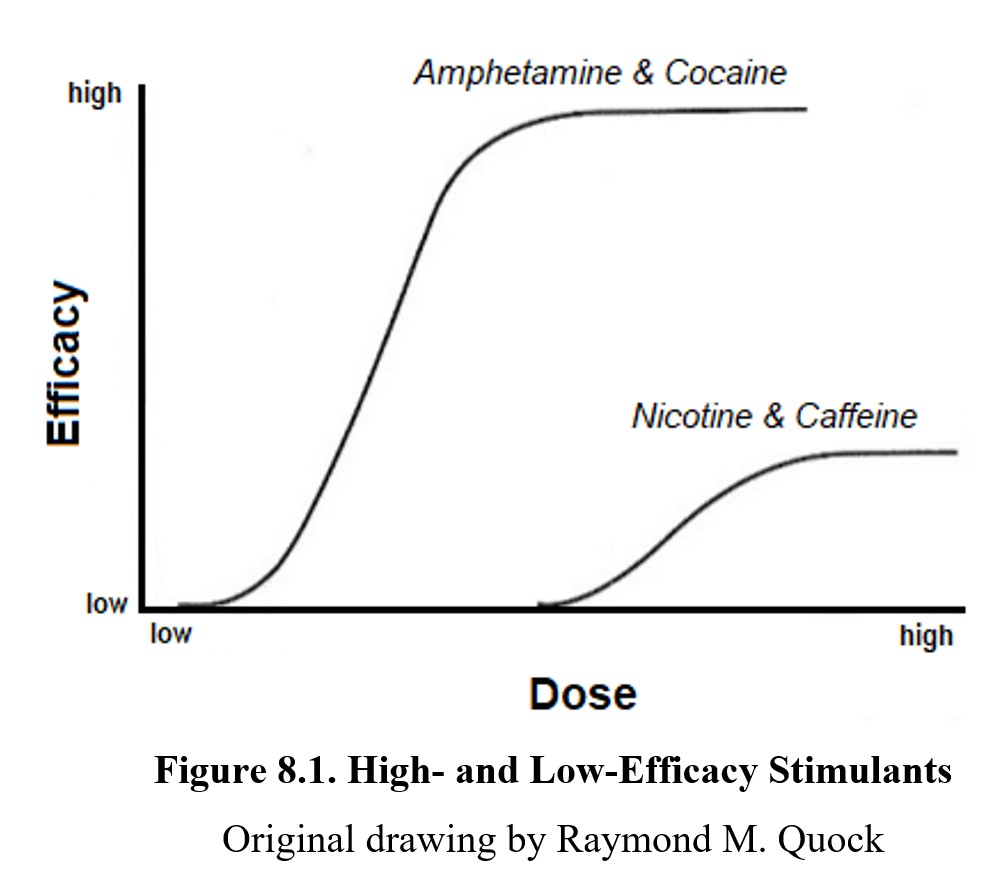
High-efficacy stimulants cause a significant increase in CNS activity. The drugs we will look at in this chapter fall into this category, as do many other sympathomimetic drugs. In comparison, low-efficacy stimulants like nicotine and caffeine produce more modest stimulation of the CNS. We will explore the differences between the two in greater depth when covering low-efficacy stimulants in the next chapter. For now, let us examine the two most notable high-efficacy stimulants or psychostimulants, cocaine and amphetamine.
8.2. Cocaine
Section Learning Objectives
- Explain the history and uses of cocaine.
- Describe the pharmacokinetic properties of cocaine and compare cocaine hydrochloride to cocaine freebase.
- Explain cocaethylene.
- Describe the pharmacodynamic properties of cocaine and the effects of long-term use.
Cocaine, often informally referred to as coke, is one of the most well-known illicit drugs. Aside from its common depictions in media, it is also the second-most used illicit drug in the U.S. behind marijuana (National Survey of Drug Use and Health, 2019). In the following section, we will explore its role in culture and medicine and outline the exact mechanisms through which it causes its stimulatory effects.
8.2.1. Drug History and Overview
Cocaine is a psychoactive substance extracted from the leaves of the coca plant, Erythroxylum coca, which grows on the eastern slopes of the Andes Mountains. Coca leaves have been chewed as a mild stimulant for thousands of years in South America. They were also chewed for medicinal purposes and as a remedy to the stress of living at high altitudes. The use of coca spread to other parts of the world with the help of Spanish conquistadors, who found its ability to make workers eat less and work longer appealing.
Chemists began to try to isolate the alkaloid responsible for these effects from the plant in the 1800s. In 1860, graduate student Albert Niemann isolated the alkaloid and named it cocaine, after the coca plant. Interest in the drug’s potential medicinal uses quickly followed. Ophthalmologist Karl Koller demonstrated its properties as a local anesthetic, while Sigmund Freud advocated for its use as a stimulant and pain reliever.
The use of cocaine was popularized throughout the late 1800s. In Europe and America, it was readily available and advertised for its therapeutic effects. The original recipe for Coca-Cola included coca leaves, hence the name. Cocaine was purchased as an over-the-counter drug up until 1916. Not long afterward, it became regulated under the Harrison Narcotics Act. By then, reports of addiction and dependence had become more common, and governments moved to regulate cocaine use.
Cocaine remains a favored anesthetic in otolaryngology because of its local anesthetic and vasoconstricting properties. For other medical and dental uses, cocaine has mostly been replaced by safer local anesthetics such as lidocaine (Xylocaine®) and procaine (Novocain®), which derive their -caine suffixes from cocaine. Clinical use of cocaine is tightly regulated as it is classified as a Schedule II controlled substance (i.e., a drug accepted for medical use with a high potential for abuse).
8.2.2. Administration and Pharmacokinetics
Cocaine, like many stimulants, comes in multiple forms. The salt form, cocaine hydrochloride (HCl), appears as a white powder that is typically snorted intranasally or dissolved and injected directly into a vein. Cocaine can be taken orally, but its effects are heavily reduced as stomach acid tends to leave it inactive, so oral administration is uncommon. It can also be used topically as a local anesthetic.
Removing the HCl from cocaine salt converts it to cocaine freebase or crack, which can be conveniently smoked. Technically, cocaine salt can be smoked as well, but this method is not popular because the resulting fumes are much more unpleasant. Cocaine freebase can be made by heating the HCl salt with volatile chemicals such as ammonia or by mixing it with baking soda in water and drying the crystals to produce the freebase cocaine. The name crack comes from the popping or crackling sound when it is heated and smoked. See the image below to compare the physical appearance of cocaine HCl (left) with that of crack cocaine (right):
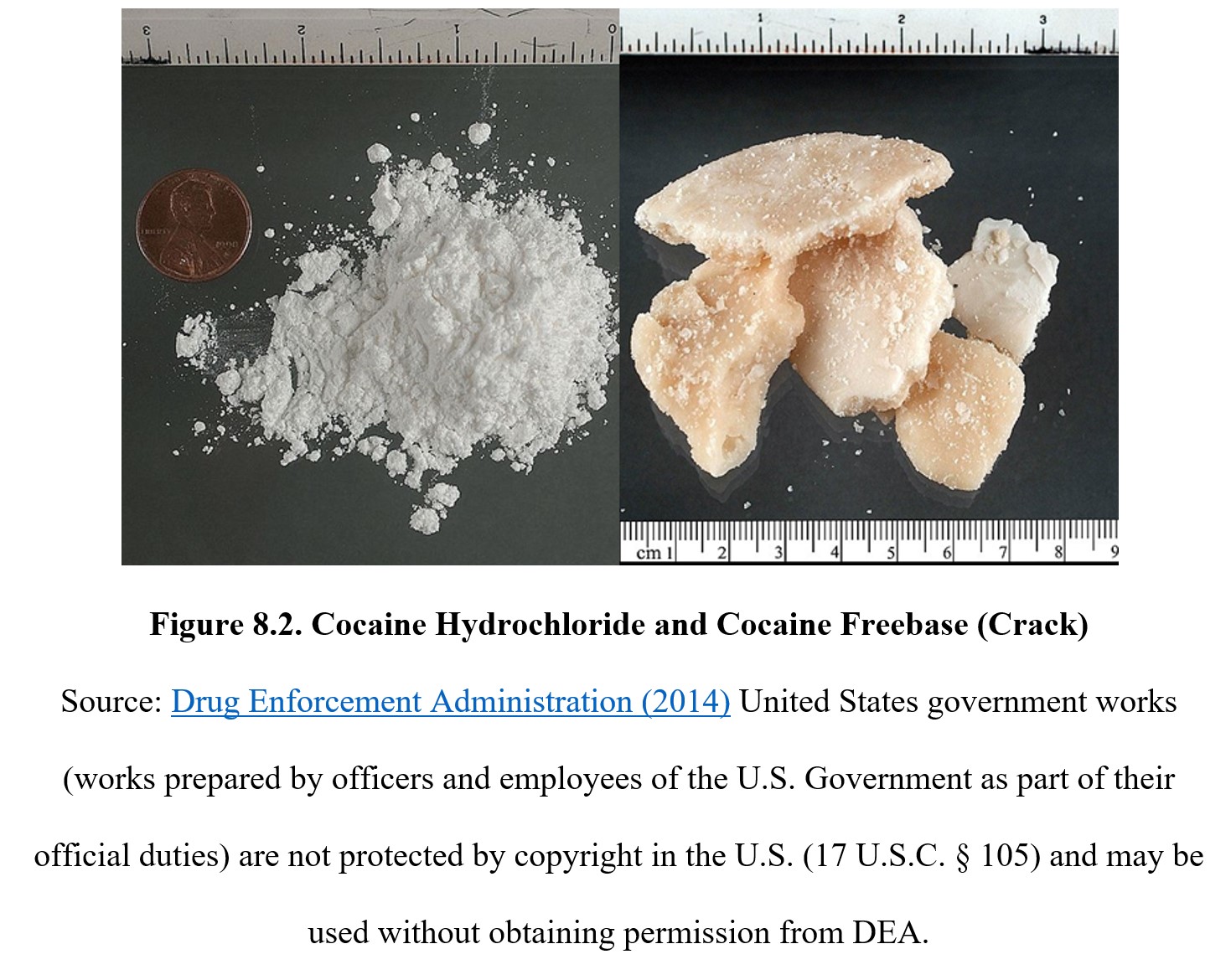
Cocaine freebase has a higher bioavailability and onset of action compared to its salt form. It also produces a more intense effect due to its increased lipid solubility and more rapid entry into the brain. As a result, smoking cocaine freebase provides a stronger and more immediate rush that is more addictive than using cocaine salt. (This should make sense to you, as in the last chapter, we discussed how the size and immediacy of a reinforcer can increase its reinforcing effect.) The Manual of Adolescent Substance Abuse Treatment calls crack the most addictive form of cocaine (Estroff, 2008). Take a look at the following chart comparing various pharmacokinetic properties of cocaine hydrochloride and cocaine freebase:
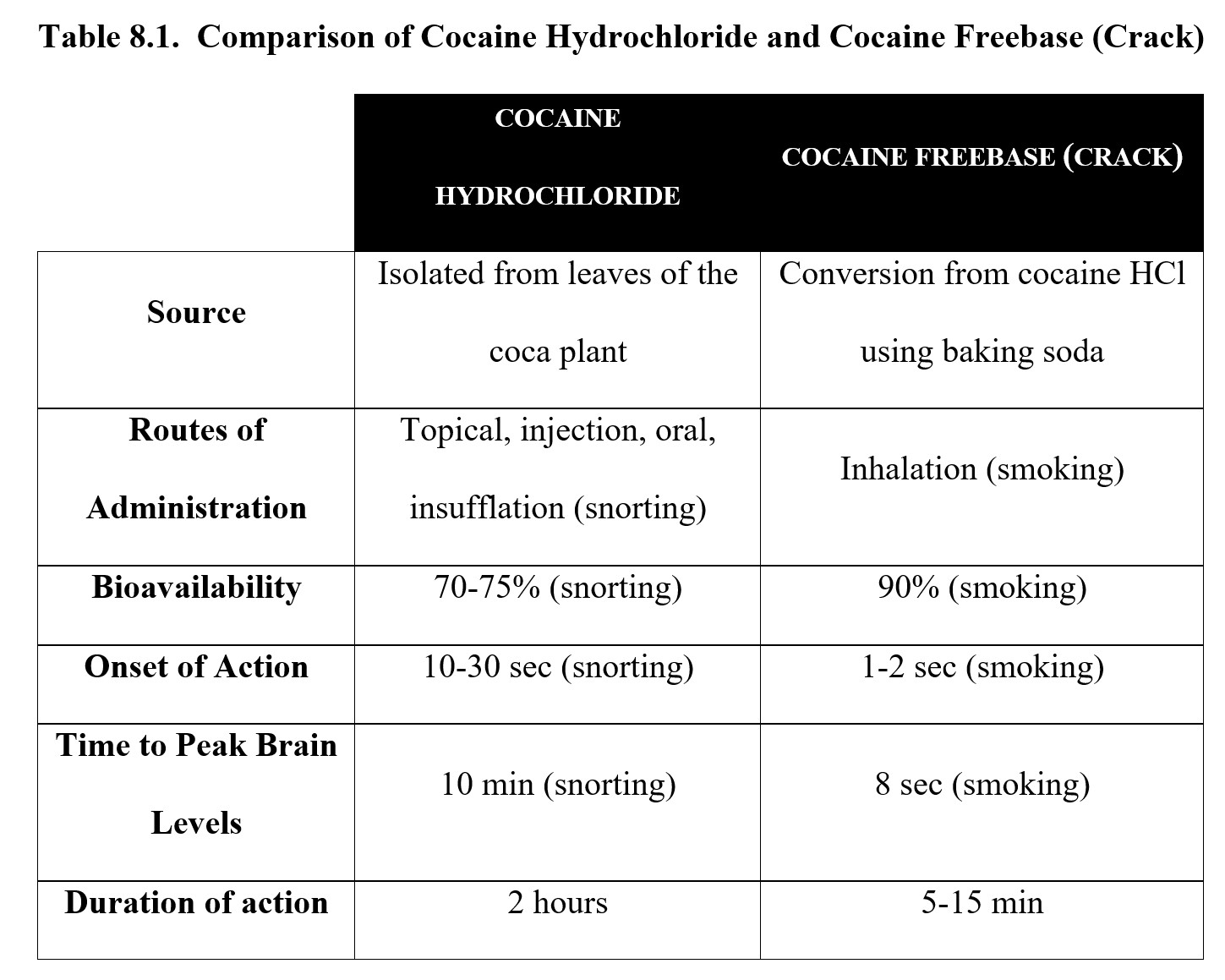
As you can see, the absorption rate depends on the route of administration. Once the drug is in the bloodstream, it is actively hydrolyzed by enzymes and has a relatively short half-life of approximately 1 hour because it is metabolized by esterase enzymes in the blood. Cocaine is capable of crossing the blood-brain barrier, which is how it reaches its neuronal targets in the CNS.
When cocaine is taken with alcohol, the two drugs interact to produce a toxic metabolite called cocaethylene in the liver, which has a lower LD50 than cocaine. Cocaethylene is psychoactive and has a similar mechanism of action to cocaine, but it has a longer half-life. Cocaethylene enhances the euphoric effects of both cocaine and alcohol, making it a synergistic interaction. But it also increases the potential toxicities of both drugs.
8.2.3. Mechanisms of Action and Effects
Cocaine produces its effects by blocking transporter proteins responsible for the neuronal reuptake of monoamines. To refresh, these are the pumps that remove the excess transmitter from the synapse and return it to the presynaptic cell. Cocaine blocks NET and DAT, which are the transporter proteins for norepinephrine and dopamine, respectively. By blocking neuronal reuptake, cocaine causes an increase in the overall amount of transmitter present in the synapse to act on postsynaptic receptors. Cocaethylene, the byproduct of cocaine and alcohol inhibits not only NET and DAT but also the serotonin transporter (SERT) so that levels of all three biogenic amines are elevated.
The cocaine-induced surge in synaptic levels of norepinephrine results in increased activation of postsynaptic alpha-adrenergic receptors in the brain. This gives rise to objective central effects of cocaine, including increased wakefulness, increased respiration, and decreased appetite (upper panel of the figure below). A buildup in synaptic levels of dopamine causes increased activation of postsynaptic dopamine receptors. This will result in subjective central effects of cocaine, including a sense of well-being (euphoria), increased sense of energy, increased sense of confidence, and, at high doses, psychotic behavior (lower panel of the figure below). Another effect of increased dopamine is the activation of the brain’s reward center which contributes to the development of physical dependence. Following the intense but short-lived stimulatory effects of cocaine, a “crash” ensues in which the subjective effects rapidly dissipate and are replaced with compensatory depression and physical exhaustion.
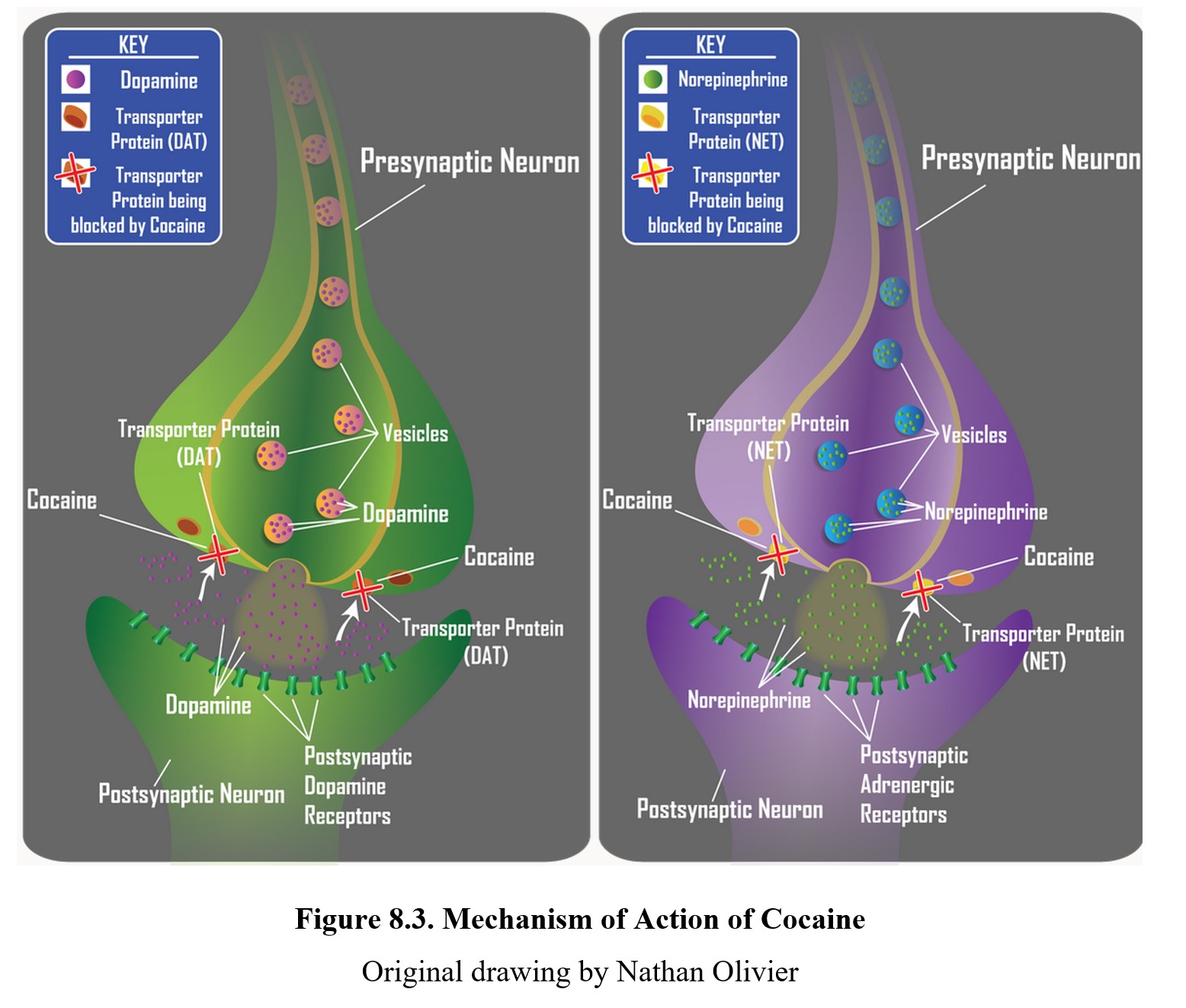
In addition to its actions in the brain, cocaine has profound physiological effects in the periphery. Inhibition of norepinephrine reuptake increases synaptic availability of the transmitter and activation of alpha-adrenergic receptors. This is responsible for the increased heart rate, vasoconstriction of blood vessels, dilation of the pupils, and other sympathomimetic effects. Excessive doses of cocaine are prone to producing cardiotoxicity, including acute myocardial infarction, heart failure, and/or cardiac arrhythmia.
Tolerance to the euphoric effects can develop over time due to downregulation (reduction in the number) of dopamine receptors. This causes the user to continually increase the dose of cocaine, which increases the risk of addiction. Cross-tolerance is also common between different stimulants that share similar mechanisms. According to researchers, tolerance to cocaine develops very rapidly over a period of days. Some scientists claims that tolerance begins after the first dose of cocaine.
Since cocaine is a psychostimulant, withdrawal signs will proceed in the opposite direction and feature depression, lethargy, dysphoria, sleep disturbance, and increased appetite. The primary withdrawal signs are drug craving, mental depression, and suicidality, which can persist for 1-2 weeks following the last use of the drug. Withdrawal signs are intensified if the polysubstance use combines abuse of cocaine with other addictive drugs.
At high doses of cocaine, people may experience psychosis and hallucinations. A common hallucination involves feeling like bugs are crawling under the skin (formication). Overdose may cause abnormally high blood pressure, excessive heart rate, or other cardiopulmonary symptoms, which may sometimes be fatal. The psychosis is consistent with the Dopamine Hypothesis of Schizophrenia, which will be discussed later in Unit 4.
It is worth noting that cocaine-induced local anesthesia is a unique drug effect not shared by other psychostimulants. Cocaine blocks sodium channels at sensory neurons, which prevents EPSPs and the generation of an action potential, thus blocking signal transmission along sensory nerves. This is a property of all drugs with local anesthetic properties, i.e., any drug with the -caine suffix. You can see this in the diagram below:
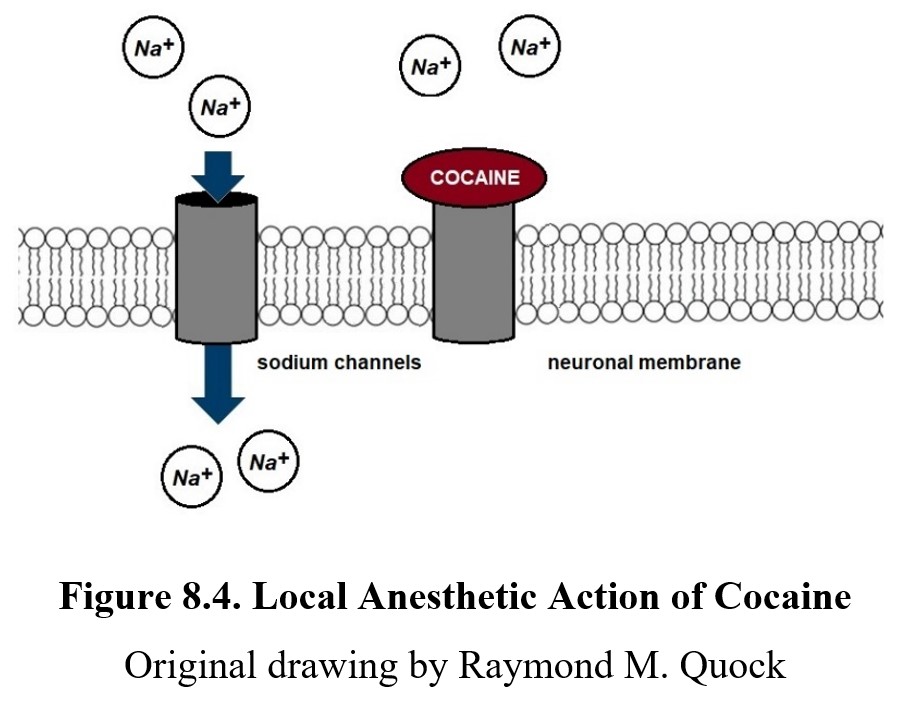
Cocaine is used recreationally as a party drug most often due to the increase in energy and euphoria that it provides. Because of this, its use often correlates with that of other party drugs such as MDMA (ecstasy) and amphetamines or other drugs such as heroin. The presence of alcohol in such environments can be particularly dangerous due to the synergistic interaction between the two mentioned earlier.
The following video is a nice summary of the pharmacological properties of cocaine.
What You Need to Know About Cocaine [4:57]
8.3. Amphetamine
Section Learning Objectives
- Explain the history and uses of amphetamine and methamphetamine.
- Define chirality and enantiomers and explain why they are pharmacologically relevant.
- Describe the pharmacokinetic properties of amphetamine and methamphetamine.
- Describe the pharmacodynamic properties of amphetamine and methamphetamine and the consequences of long-term use.
Amphetamine is another major stimulant that sees therapeutic and recreational use. Its chemical structure is the basis for substituted amphetamines, which are drugs that have similar structures to amphetamine and include cathinone and MDMA. The most notable of these is methamphetamine (often called meth or “speed”). It is an even more potent psychostimulant than amphetamine itself. We will focus on amphetamine and methamphetamine in this section, but keep in mind that some other drugs like methylphenidate and cathinone can produce similar effects.
8.3.1. Drug History and Overview
Amphetamine was first synthesized in 1927 by chemist G. A. Alles, who was searching for a substitute for ephedrine to treat asthma and allergies. Ephedrine is a psychoactive ingredient found in numerous species of Ephedra plants, most notably má huáng in Asia, where it has been used in Chinese medicine for thousands of years.
Amphetamine was initially marketed under the name Benzedrine® as a treatment for narcolepsy, obesity, mild depression, and various other medical conditions. Amphetamines were soon seen as being capable of enhancing cognition, which led to their widespread use in academics in the late 1930s. It was also given to World War II bomber pilots and sentries as energy pills to increase wakefulness and reduce fatigue and appetite. The potential for addiction was largely ignored until after the war when addiction rates soared and governments around the world started to regulate amphetamines more strictly.
Currently, amphetamine is classified as a Schedule II controlled substance, the same as cocaine. The accepted medical use for amphetamine that keeps it out of Schedule I is its use in treating attention deficit hyperactivity disorder (ADHD). This was one of the earliest therapeutic applications discovered for amphetamine, and it has remained one of the primary options in treating ADHD to this day. That said, the exact formulation used to treat ADHD has changed since the 1930s.
Left-handed molecules: Chirality and enantiomers
Are your right and left hands identical? They are certainly very similar. In fact, they are mirror images of each other. Despite this, they are not identical. You will always be able to discern a right hand from a left hand because hands are not symmetrical—the thumb is on a different side for each.
In a similar fashion, certain molecules can be mirror images of each other without being perfectly identical. These molecules are chiral (from cheir in Greek for “hand”), meaning they have right-handed and left-handed versions. You can see an example of a chiral molecule in the figure below. In the panel to the left, note that the two structures are the mirror images of one another. In the panel to the right, see how the structure of the L-enantiomer is compatible with sites of binding on the receptor. But the D-enantiomer is not compatible with the sites of the receptor. Therefore, only the L-enantiomer is able to bind to and activate the receptor.
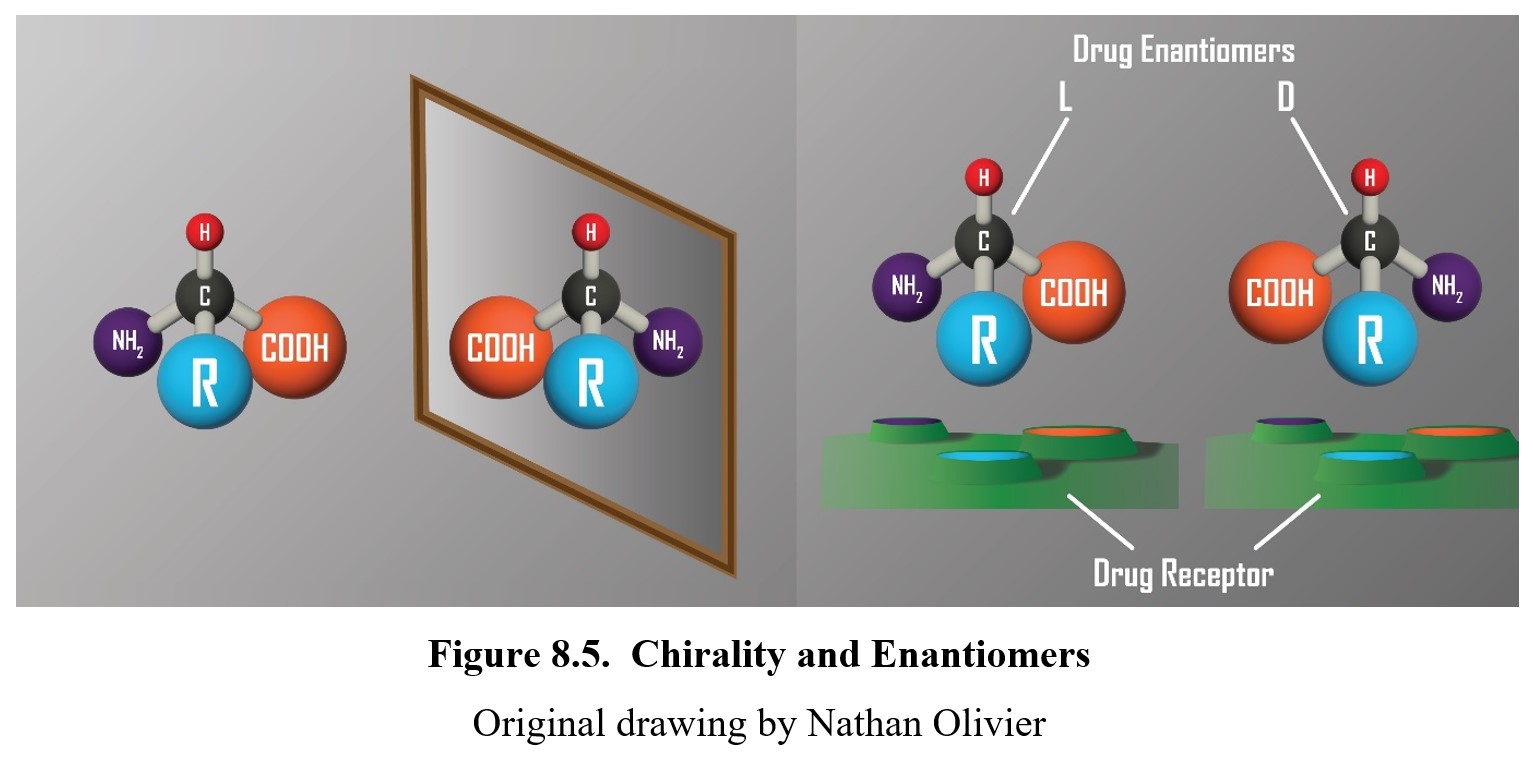
What does this have to do with amphetamine? Well, amphetamine is actually a chiral molecule. It has right-handed and left-handed versions called enantiomers. The right-handed version is called dextroamphetamine or D-amphetamine, while the left-handed is levoamphetamine or L-amphetamine. (This naming scheme might sound familiar—in fact, we’ve already mentioned a chiral molecule before called L-DOPA, the precursor to dopamine. As you would expect, there is a right-handed version of it called D-DOPA instead.)
The reason why enantiomers are important is that they sometimes have different pharmacological properties. D-amphetamine is more centrally active, while L-amphetamine is more peripherally active. So, for a drug like amphetamine, it is important to know how much of each type is present in the mixture.
The original formula for amphetamine had equal parts of L- and D-amphetamine, which is called a racemic mixture. (You don’t need to know this term for this class, but it might show up when doing further research.) Later, a drug known as Dexedrine® was developed using only the more potent D-amphetamine enantiomers. The modern amphetamine used to treat ADHD which you are probably most familiar with is Adderall®, which is a 3:1 mixture of D-amphetamine to L-amphetamine. Another popular stimulant, methylphenidate (Ritalin®, Concerta®), is also used to treat ADHD. This will be covered in more detail in a later chapter on ADHD.
Amphetamines are also approved by the FDA for treatment of narcolepsy, a chronic sleep disorder featuring sudden sleep attacks during times that are normally inconducive to sleep. Stimulants can ward off sleep attacks and allow persons to remain awake during the day. At one time, amphetamines were widely used to treat obesity. However, this use is no longer approved by the FDA due to development of tolerance to the anorectic effect of amphetamines and the need to progressively increase drug dose, leading to an exacerbated risk of physical dependence.
Amphetamine vs. Methylphenidate [3:14]
Methamphetamine is also an approved medication for ADHD and treatment-resistant obesity. However, there are safer alternatives with less toxicity and a lower potential for developing physical dependence. Because of this, there is only one legal prescription form of methamphetamine (Desoxyn®) that cannot be refilled. As such, the most common use of methamphetamine is illicit for recreational purposes. Its limited accepted medical uses is still enough for methamphetamine to be classified as a Schedule II drug. That said, its prescription and use are tracked much more intently than amphetamine.
One consequence of the abuse potential of methamphetamine is the limitations placed on the distribution of pseudoephedrine, a substance found in the same plants as ephedrine. It is an active ingredient in certain decongestants (e.g., Sudafed®), but, because of its chemical structure, it can be used to illegally manufacture methamphetamine. Consequently, decongestants containing pseudoephedrine that used to be sold as over-the-counter medicines are now more tightly regulated, with requirements for photo ID and limits on purchase quantity. Illicit production of methamphetamine overseas also involves conversion of pseudoephedrine or a precursor to pseudoephedrine.
8.3.2. Administration and Pharmacokinetics
For therapeutic use, amphetamine is typically given orally, which allows for consistent blood concentrations over time. Amphetamine can also be administered via injection, inhalation, or intranasally, which results in more rapid absorption. The rate of absorption is also dependent on the ionization of the drug. Amphetamine is a weak base that is commonly ionized in the acidic environment of the stomach, which contributes to the slow rate of oral absorption.
Once amphetamine reaches the bloodstream, it is readily distributed throughout the body and can easily cross the blood-brain barrier. Some amphetamine (about 20%) is bound to plasma proteins. Amphetamine and methamphetamine have much longer half-lives than cocaine. The half-life is typically around 10-12 hours and changes depending on the pH level and mixture of enantiomers. In addition, the effects last longer. The high from smoking cocaine tends to last 20 to 30 minutes, while the high from methamphetamine can last from 8 to 24 hours. Ultimately, amphetamine and methamphetamine are both metabolized by enzymes in the liver.
Part of the reason for the increased potency and effects of methamphetamine is due to the fact that one of its active metabolites is amphetamine itself. Thus, to eliminate methamphetamine from the system, it must first be metabolized into amphetamine, which in turn must be metabolized as well. The amphetamine produced as a metabolite helps to prolong the effects of methamphetamine.
8.3.3. Mechanisms of Action and Effects
Similar to cocaine, amphetamine increases synaptic levels of monoamines such as norepinephrine, dopamine, and serotonin. Amphetamine has a similar chemical structure to monoamines, so instead of blocking transport proteins, amphetamine molecules are actually mistakenly taken into the axon terminal by the reuptake mechanism.
Once inside the nerve terminal, amphetamine increases the release of monoamines while also forcing the transporter proteins to work in reverse. Instead of pumping neurotransmitters out of the synapse, more and more neurotransmitters are pushed into the synapse. The figure below shows amphetamine-induced release of norepinephrine in the left panel and dopamine in the right panel. In addition, amphetamines have been found to also inhibit monoamine oxidase, the enzyme responsible for breaking down monoamine transmitters that have been taken back up by the presynaptic cell. But this is a secondary effect that has been reported, but the main mechanism of action is induction of neuronal release of transmitter.
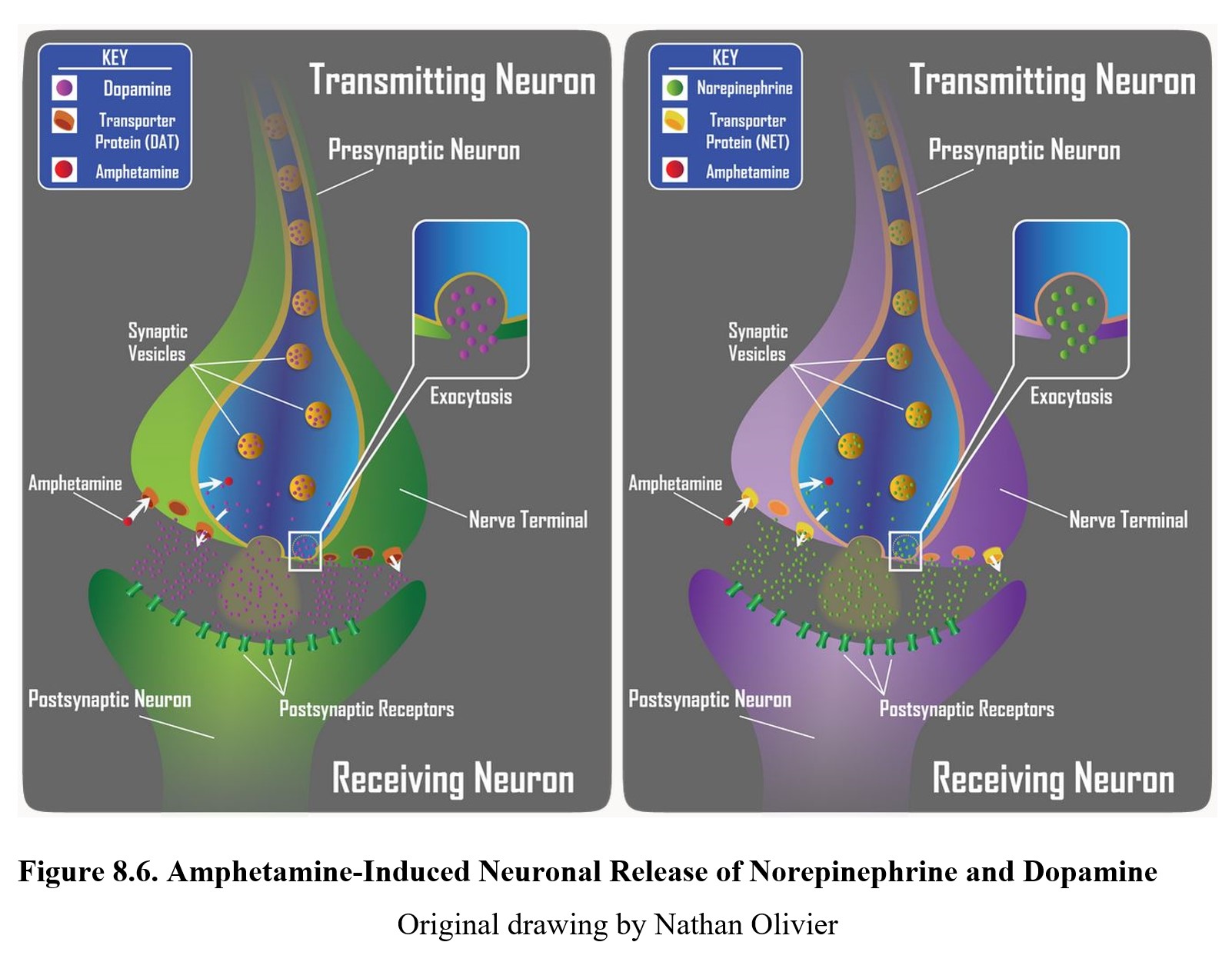
The neurotransmitters released by amphetamine activate the same alpha-adrenergic and dopaminergic receptors that cocaine does, leading to similar stimulating effects and euphoria. Methamphetamine has a similar mechanism of action but has a stronger effect on the dopamine transporter, which accounts for its greater addictive properties.
Because of its spectrum of pharmacological effects, amphetamines can be misused for a variety of different purposes. Common reasons include weight loss, pleasure-seeking, increased focus, enhanced cognition, or enhanced sports performance. Amphetamines are among performance-enhancing drugs (PED) that are banned by the Olympics and other international and professional sports.
The tolerance and withdrawal symptoms are also very similar to those of cocaine since they target similar receptors. High doses can result in some of the cardiopulmonary symptoms seen with cocaine intoxication, but changes in mental state such as anxiety, paranoia, and hallucinations are more common with amphetamine and methamphetamine. Physical effects of amphetamine overdose include hypertensive emergency, tachycardia, and cerebrovascular accident (stroke).
Amphetamines are weak bases. The degree of ionization can be influenced by manipulation of the urinary pH. Amphetamine overdose can be managed by acidification of the urine with ammonium chloride. The decrease in urinary pH will increase the number of amphetamine molecules that are ionized. This reduces the amount of amphetamine that will be reabsorbed from the renal tubes and back into the bloodstream. Consequently, excessive amphetamine in the body will be eliminated in the urine.
If acidified urine increases the ionization of amphetamine and hastens its elimination in the urine, you can predict the consequence if the urine were to be more alkaline or basic. If the urinary pH is higher, amphetamine will be less ionized and be more readily absorbed across membranes. Hence, someone who self-medicates with sodium bicarbonate for indigestion or consumes large amounts of meat or certain fruits or vegetables may experience signs of amphetamine toxicity because its elimination is impaired. A good mnemonic to keep this straight is: Weak acids are better absorbed in acid, while weak bases are better absorbed in base.
Methamphetamine, also referred to as speed, meth, or crystal meth, has a similar mechanism of action to amphetamine but has a stronger effect on the dopamine transporter, which accounts for its greater addictive properties. The following is a summary of salient facts about meth:
Methamphetamine (Meth) Drug Facts [3:40]
The tolerance and withdrawal symptoms are also very similar to those of cocaine since they target similar receptors. High doses can result in some of the cardiopulmonary symptoms seen with cocaine intoxication, but changes in mental state such as anxiety, paranoia, and hallucinations are more common with amphetamine and methamphetamine. Physical effects of amphetamine overdose include hypertensive emergency, tachycardia, and cerebrovascular accident (stroke).
Long-term use of methamphetamine is associated with numerous health risks. “Meth mouth,” or permanent degradation of teeth, can occur due to the side effects of methamphetamine such as dry mouth, increased grinding of teeth (bruxism), increased consumption of sugary beverages, and poor oral hygiene.
Shocking Effects Of Meth Mouth [3:59]
In addition, methamphetamine also results in an increased production of a lipid molecule, ceramide, which speeds up cell metabolism and results in earlier cell death and faster aging (see Fig. 8.7 below). This can cause long-term health problems and permanent damage.
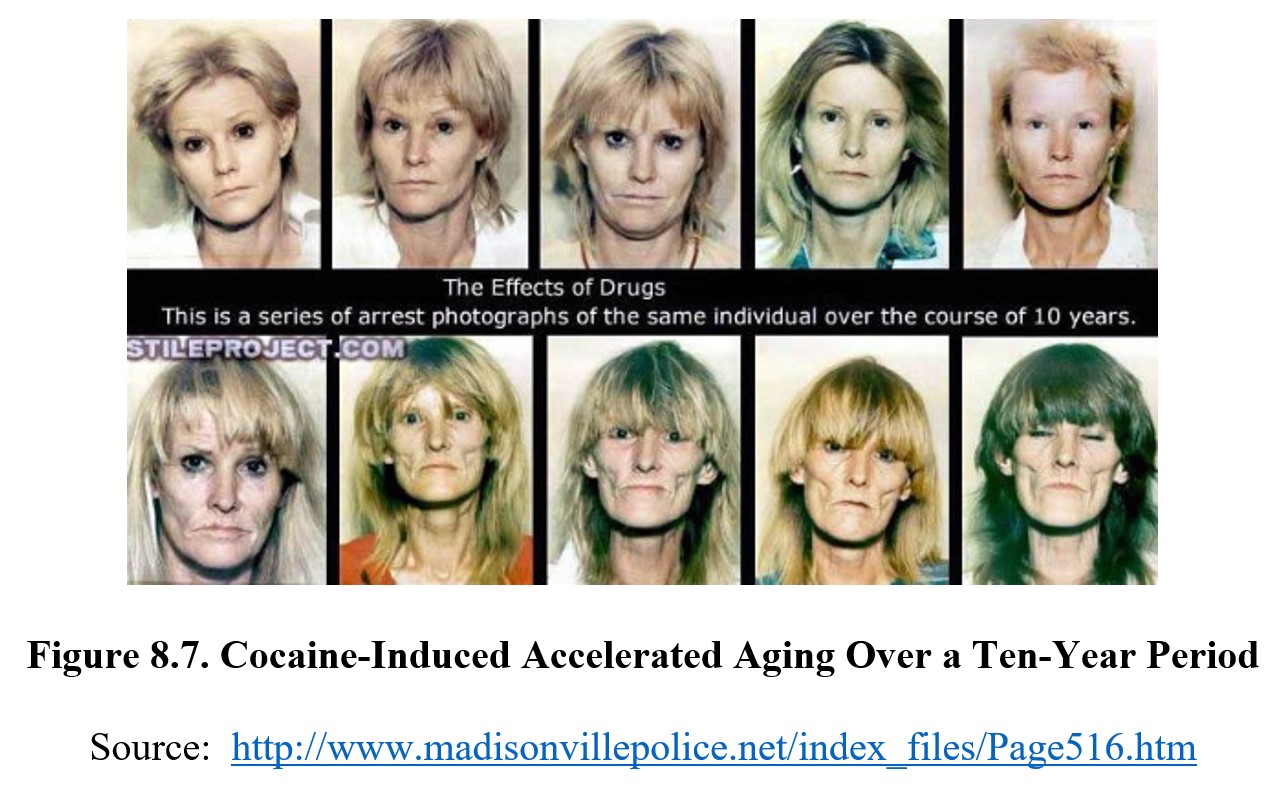
Chapter Summary and Review
In this chapter, we introduced our first class of drugs, stimulants, and took a detailed look at two key high-efficacy psychostimulants: cocaine and amphetamine. We documented the history, uses, and pharmacological properties of both drugs. This involved distinguishing between cocaine hydrochloride (cocaine salt) and cocaine freebase (crack cocaine) and describing the interaction between cocaine and alcohol. We also discussed chirality in the context of amphetamine formulas and examined methamphetamine, a potent substituted amphetamine that shares similar mechanisms of action. Cocaine and amphetamine produce similar drug effects but differ in their mechanisms of action and pharmacokinetic characteristics.
In the next chapter, we will move on to low-efficacy stimulants. As mentioned before, these chapters will draw from all of the topics we have covered previously. Therefore, if you notice yourself stumbling or having difficulties with a particular concept, it is not too late to go back and review or seek help.
Chapter 8 Practice Questions
Answer the following:
- What class of neurotransmitters do sympathomimetic drugs target? Why?
- What is the therapeutic use for cocaine, and how does it achieve this effect?
- Compare the pharmacokinetics of cocaine hydrochloride and crack cocaine.
- What is the bioavailability of crack cocaine when smoked?
- What metabolite forms when cocaine and alcohol interact? Why is it dangerous?
- Why is it important to be aware of enantiomers when developing a new drug?
- Describe the mechanisms of action for cocaine and amphetamine. In what ways are they the same? How are they different?
- Describe the cause of “meth mouth”.
- What is responsible for accelerated aging?
2nd edition