Chapter 4: Neurotransmission
2nd edition as of August 2022
Chapter Overview
In the previous chapter, we learned how electrical signals called action potentials to propagate through neurons. At the end of the chapter, we briefly mentioned how a single neuron can transfer a signal to a post-synaptic neuron by releasing certain chemicals into the synapse and activating postsynaptic receptors. The focus of this chapter is on these chemicals, what they are, how they are released, and how they can alter cell physiology.
In this chapter, we will explore the process of neurotransmission: how it was discovered, how it works, and how drugs can interact with the process. We will then cover several neurotransmitters and receptors to see examples of what effects they can have on human functioning. Just like the chapter on the nervous system, many terms are introduced that will be used throughout the course, so make sure to practice and test yourself until you are comfortable with the terminology.
Chapter Outline
4.1. Overview of Neurotransmission
- 4.1.1. The Discovery of Neurotransmitters
- 4.1.2. The Process of Neurotransmission
- 4.1.3. Ligands and Receptors
- 4.1.4. How Drugs Alter Neurotransmission
4.2. Neurochemical Transmitters and Receptors
- 4.2.1. Acetylcholine
- 4.2.2. Norepinephrine, Epinephrine, and Dopamine
- 4.2.3. Serotonin and Histamine
- 4.2.4. Glutamate, GABA, and Glycine
- 4.2.5. Endorphins and Substance P
- 4.2.6. Nitric Oxide
- 4.2.7. Transmitters and Receptors Review
Chapter Learning Outcomes
- Describe how neurotransmission occurs.
- Define ligand and outline major receptors in the body.
- List and describe the various neurotransmitters to include their general functions, and the receptors they activate.
4.1. Overview of Neurotransmission
Section Learning Objectives
- Describe the concept of neurochemical transmission, how it was discovered, and why it is important.
- Explain the process of neurotransmission and define all relevant terms.
- Differentiate between ionotropic and metabotropic receptors and describe ligand affinity and efficacy.
- Explain how drugs can influence function by altering neurotransmission.
By now, you know that neurotransmitters are chemical substances that are released into the synapse once an action potential reaches an axon terminal. As the name suggests, it transmits a signal across a synapse, though you may be wondering why? Why bother with synapses and neurotransmitters at all? Why not just have the neuron transmit the signal directly to the target cell, be it a neuron or a non-neuronal cell?
It is certainly not an in1tuitive idea but using chemicals to transmit the signal indirectly, comes with distinct advantages. This section is meant to not only explain what neurotransmission is, but also why it occurs in the first place. Perhaps most importantly, how drugs can influence it.
4.1.1. The Discovery of Neurotransmitters
How exactly did the idea come about? By the 20th century, scientists knew that nerve fibers would come into close proximity to target cells but were still uncertain how the signals actually reached the target. It could have been direct electrical contact since electrical stimulation of muscle could stimulate muscle contraction. But certain chemicals could achieve similar effects. There was great disagreement, but with the technology of the time, there was no easy way to determine which method was used by neurons to deliver their signal to target cells.
The person who would finally settle the debate was a German pharmacologist named Otto Loewi. In 1921, he came up with an experiment that conclusively proved neurochemical transmission between the transmitting neuron and the target cell. His experiment used two isolated frog hearts in adjacent tissue baths. For the first heart (shown on the left), Loewi stimulated the vagus nerve (cranial nerve X), which was still attached to the heart. This resulted in a slowing of the heart rate. This was already recognized by physiologists at the time. Loewi then took fluid from the first tissue bath and applied it to the second tissue bath, in which the second heart was denervated, i.e., its vagus nerve was removed. This caused the heart rate to slow in the second heart as well. Because only the fluid was transferred, it ruled out electrical conduction—there had to be some chemical substance in the fluid that carried the signal.
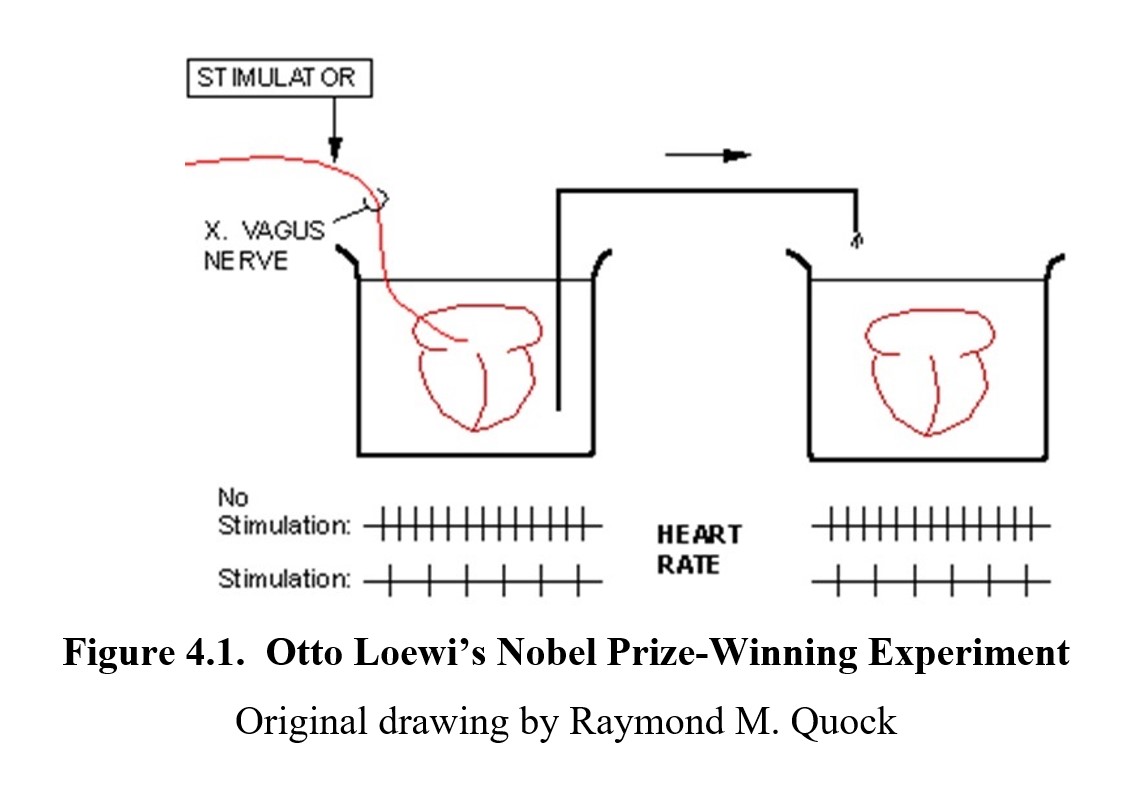
Loewi called this substance vagusstoff, literally meaning “vagus substance” in German. This experiment was the first scientific evidence to show that neurons communicated over the synapse chemically rather than electrically. Vagussoff was subsequently shown to be acetylcholine, the first neurotransmitter to be identified. Loewi published his results and would later win the Nobel Prize in Physiology or Medicine in 1936 for his discovery. The Nobel co-winner was the English physiologist Sir Henry Dale, who described many of the effects of acetylcholine.
Let us briefly review what we know about synaptic transmission before we discuss specific neurotransmitters and their receptors.
The Nervous System, Part 3 – Synapses! [10:56]
4.1.2. The Process of Neurotransmission
To understand the process, let us return to the synapse. In the previous chapter, we mentioned that when an action potential reaches an axon terminal, it causes the terminal to release certain chemicals. In a resting neuron, these neurotransmitters are stored in little bubbles within the cell. The bubbles are made out of the same material as the cell membrane and are called synaptic vesicles. You can see them in the diagram below:
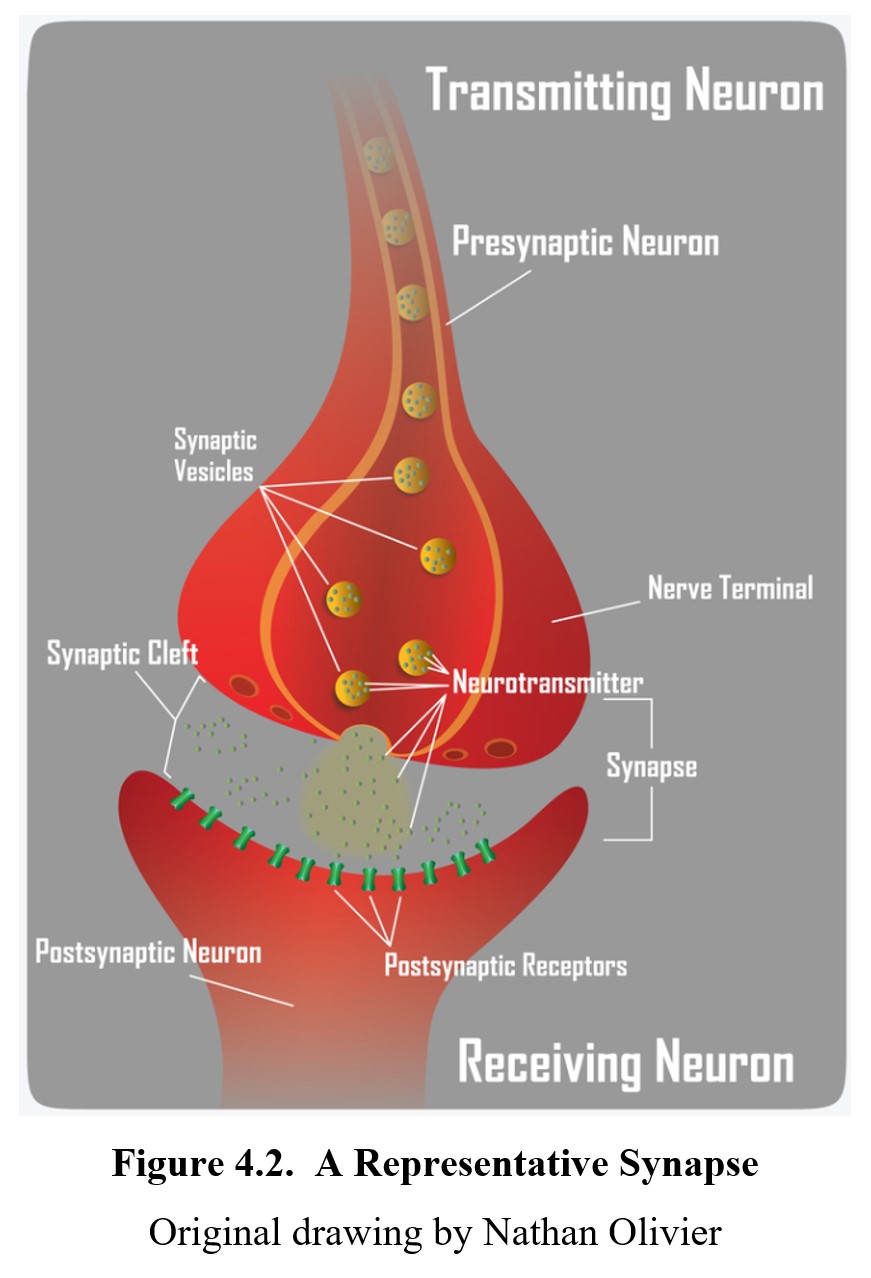
Even when the neuron is at rest, there is a spontaneous release or leakage of a small amount of neurotransmitter into the synaptic cleft. The amount is insufficient to cause a post-synaptic potential, though, and most of the neurotransmitter remains in the vesicles. To release enough of the neurotransmitters to trigger a response, an action potential must reach the axon terminal.
Let us go over each step covered in that video in more detail. When an action potential arrives at the terminal, various voltage-gated ion channels are opened. Along with the usual sodium and potassium ion channels, calcium ion (Ca2+) channels are opened. Calcium ions flow into the cell and cause the synaptic vesicles to migrate towards the cell membrane and fuse together causing the release of the stored neurotransmitter into the synaptic cleft.
Neurotransmitters travel across the synapse and activate postsynaptic receptors, which are protein structures that respond to neurotransmitters. The type of response depends on the type of receptor. The simplest is a sodium ion channel that opens when activated, allowing sodium ions into the post-synaptic neuron, depolarizing it, and generating an EPSP. Another example is the GABAA chloride ion channel receptor complex. In response to GABA and various drugs, the chloride channel opens permitting an influx of chloride ions, which causes hyperpolarization and IPSP.
Eventually, the neurotransmitters are released from the receptors and return to the synaptic cleft. At this point, they need to be removed from the cleft somehow; otherwise, they would continue to activate receptors. Most neurotransmitters are returned to the presynaptic cell by transporter proteins in a process called neuronal reuptake or, simply, reuptake. Once returned to the cell, they are repackaged into vesicles for recycling or destroyed by enzymes. A few neurotransmitters are destroyed in the synaptic cleft instead (e.g., acetylcholine).
4.1.3. Ligands and Receptors
Although we have been talking about how neurotransmitters can bind to and activate receptors, not every chemical that can do so is a neurotransmitter. Instead, we use the more general term ligand to refer to any chemical that can bind to a receptor. Ligands can occur naturally in our body (as in the case of neurotransmitters and hormones) or be introduced from outside (like certain types of drugs). We use the term endogenous to refer to the former and exogenous to refer to the latter. (The prefixes endo- and exo- mean inside and outside, respectively.) Exogenous ligands, on the other hand, are not naturally occurring in the body but include drugs originating from plants or pharmaceutical companies.
When looking at receptors, there are two major types in the human body. The first are ionotropic receptors. As you can probably guess by the name, they have something to do with ions. The suffix –tropic in this context means “affecting,” so these are receptors that affect ion channels. Another name for them is ligand-gated ion channels because the channels can be opened or closed by ligands. (Compare this with the voltage-gated ion channels that create action potentials.) In the following figure, the sodium channel on the left is closed, and sodium ions do not enter the cell. In the sodium channel on the right, an excitatory ligand binds to the active site and opens the sodium gate. The activated sodium channel now allows an influx of sodium ions into the cell. The following article highlights the differences between these two types of receptors.
Difference Between Ionotropic and Metabotropic Receptors
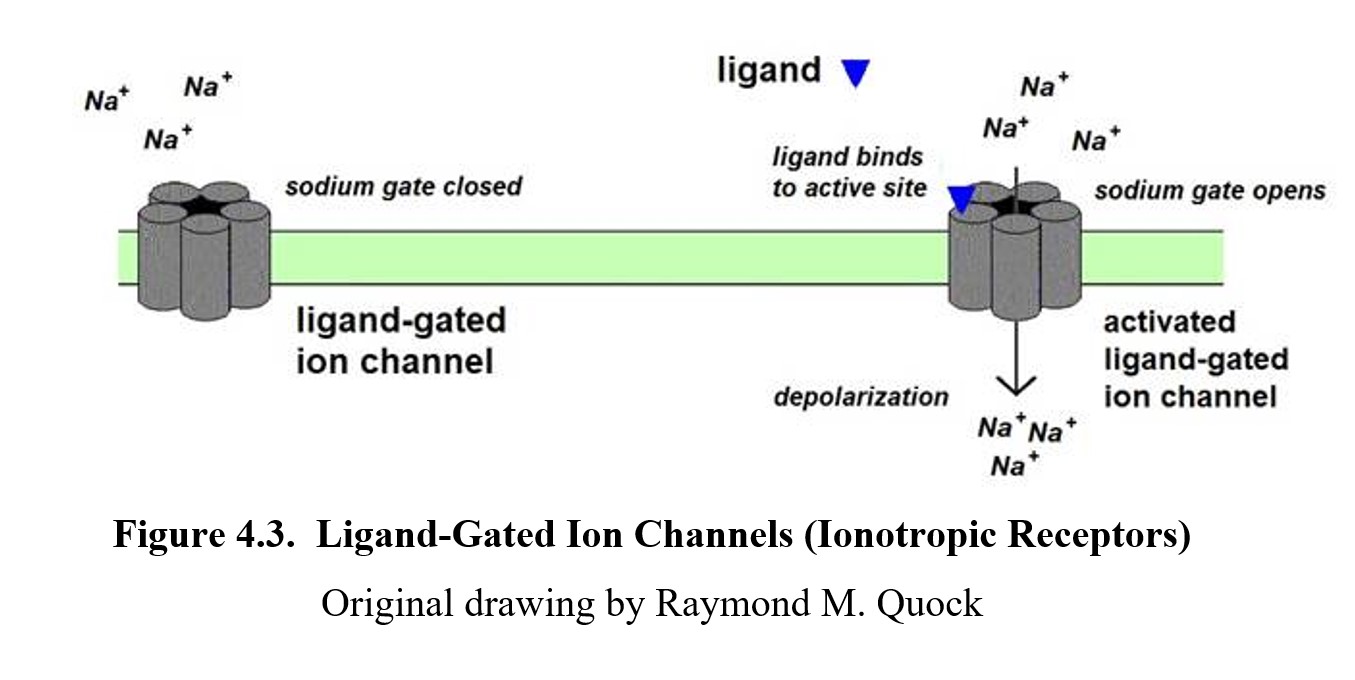
The results of the ligand-receptor interaction depend on the type of ion channel and whether the ligand is excitatory or inhibitory. Sodium ion (Na+) channels depolarize the postsynaptic neuron, while chlorine channels (Cl-) hyperpolarize it. Ionotropic receptors tend to be very fast-acting. They activate almost immediately after the neurotransmitter binds to them, and they close quickly once the neurotransmitter is removed from the synaptic cleft.
The second type of receptor is the metabotropic receptor, which is more populous than ionotropic receptors. The metabo- prefix is the same one found in words like metabolism, and both refer to chemical processes. In contrast to ionotropic receptors, they open ion channels indirectly through secondary messengers. These messengers communicate with other parts of the cell through a series of steps or cascades, eventually opening or closing ion channels. You can see this in the following diagram (compare with the ionotropic one):
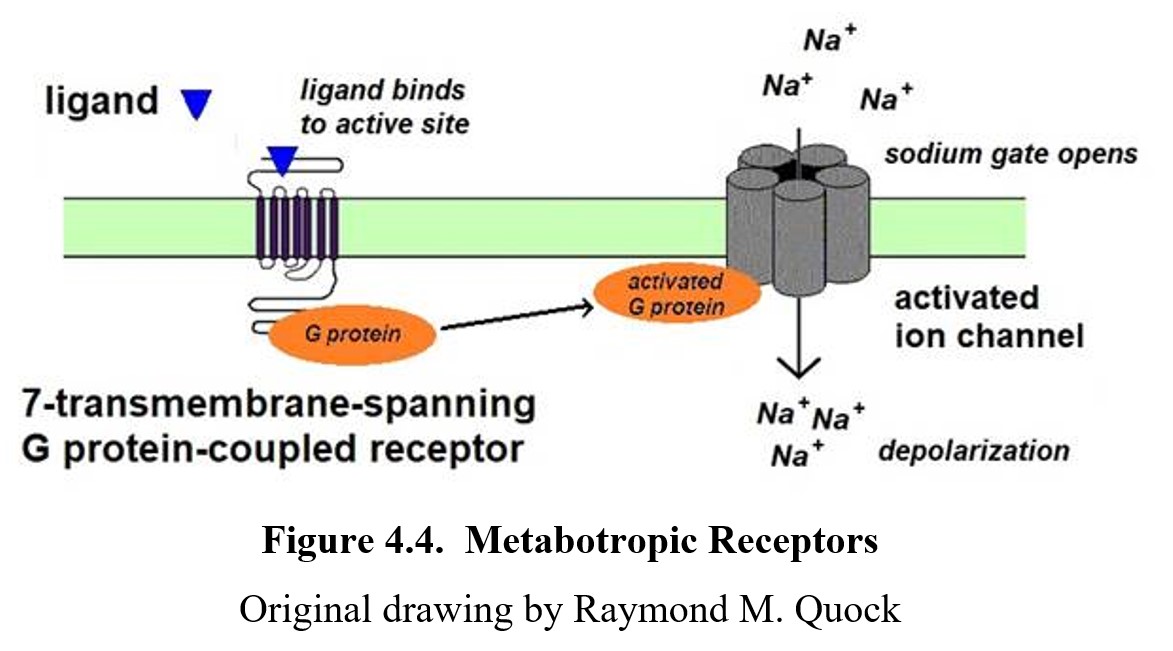
As you can see, the neurotransmitter does not directly bind to the ion channel but instead binds to the metabotropic receptor, which then opens the ion channel through a series of steps. Due to the cascade of steps, metabotropic receptors are slower to respond than ionotropic receptors. But because the secondary messengers can change other aspects of the cell’s physiology, the effects (depolarization or hyperpolarization) can last longer and be more widespread.
One type of metabotropic receptor worth noting is the G-protein coupled receptor (GPCR) for short. These receptors use G-proteins as messengers, hence the name. They are involved in many important pathways and are often the target of therapeutic drugs as a result. Around 34% of all FDA-approved drugs target GPCRs (Hauser et al., 2018). They are also known as seven-transmembrane (7TM) receptors because they consist of long chains of amino acids (polypeptides) that snake across the cell membrane seven times.
The following is a short video that summarizes the differences between inotropic and metabotropic receptors:
Ionotropic and Metabotropic Receptors [2:53]
Something important to remember is that it is the receptor, not the neurotransmitter, that determines the function. The same neurotransmitter (or ligand) can cause different effects if it binds to different receptors. Additionally, ligands and receptors are not always a perfect fit. Some ligands can bind to receptors more easily than others. We would say that those ligands have a high affinity for that receptor. But just because a ligand binds to a receptor does not mean it activates it. A ligand’s ability to activate the receptor is its efficacy. It is possible to have ligands with a high affinity but low efficacy, meaning they easily bond to a receptor but do not activate it. Indeed, this is how certain drugs work, by blocking certain receptors and preventing them from being activated. This is analogous to a key fitting into a lock (affinity) and having the appropriate ridges and cuts to open the lock (efficacy).
4.1.4. How Drugs Alter Neurotransmission
As mentioned at the start of this section, drugs influence the body by altering neurotransmission. We will save the exact methods for when we discuss individual drugs, but you should be able to tell that there are many ways this can occur. Some drugs mimic endogenous ligands, activating receptors directly; others interfere with reuptake, and some block receptors by binding to them without activating them.
How the drug alters neurotransmission determines how our behavior changes. Often, learning the effects of a drug starts with learning how it affects neurotransmission. Once you know that, you will be able to predict the kinds of physiological changes that will occur while under the influence of the drug. This pattern will show up with every type of drug we cover in this class, so it is a good idea to get comfortable with it.
4.2. Neurochemical Transmitters and Receptors
Section Learning Objectives
- Provide examples of neurochemical transmitters, their general functions, and the receptors they activate.
- Explain the classification of various types of neurotransmitters, including monoamines, amino acids, peptides, and gaseous signaling molecules.
Now that you know how neurotransmission occurs, it is time to learn about some of the neurotransmitters that are used in the human body. There are many more neurotransmitters than what is included in this section, possibly up to one hundred. The ones selected in this section are some of the most prevalent and well-researched neurotransmitters. Most of these will be affected in some way by the drugs that we will examine later in the course.
There is a lot of material in this section, yet you should not worry about it. At the end of the section, there will be a table to give you an overview of all the important information. As you read this section, try to associate each neurotransmitter with its function. Once you reach the end, you will be able to go over all the receptors and categories again and organize them in your thoughts.
4.2.1. Acetylcholine
In this subsection, we will look at acetylcholine, the first neurotransmitter to be identified. This was the chemical involved in Otto Loewi’s experiment that he named vagusstoff. Acetylcholine had already been discovered in biological organisms, and Loewi and other researchers suspected that vagusstoff was acetylcholine, although it took a few years before this was verified.
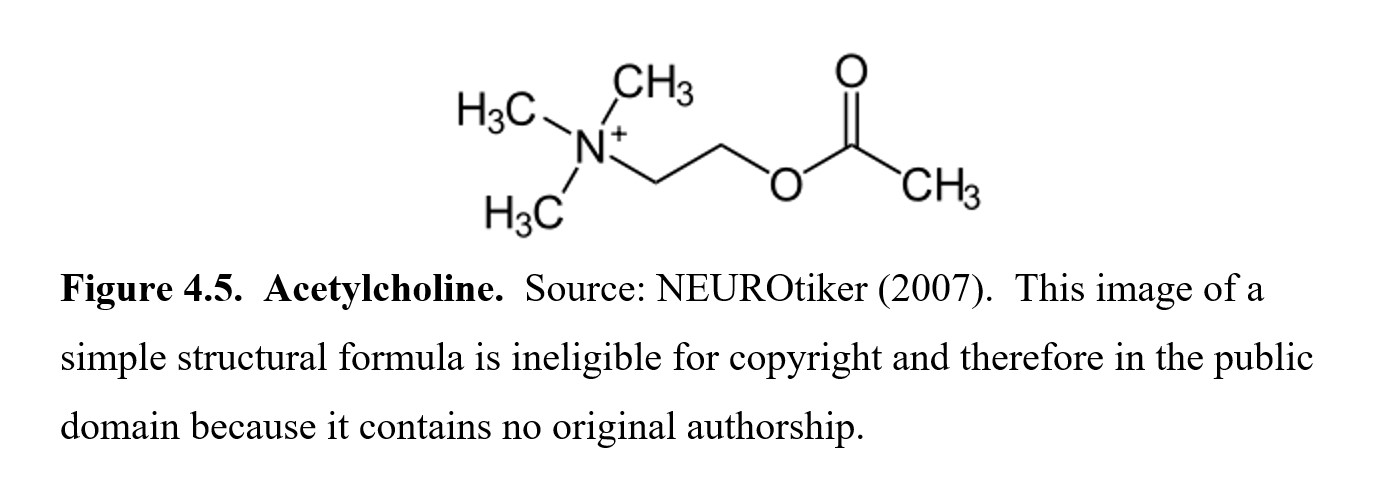
In the PNS, acetylcholine plays a large role in the parasympathetic nervous system. Recall that in Loewi’s experiment, the vagusstoff caused the heart rate to slow down. This is one of the many effects that acetylcholine is responsible for, along with other “rest-and-digest” responses such as pupil constriction, digestion, and micturition (urination). Acetylcholine is also the neurotransmitter that activates skeletal muscle in the somatic nervous system, meaning your voluntary movements are all regulated by this neurotransmitter.
In the CNS, acetylcholine plays an important role in attention, arousal learning, and memory among other functions. In the hippocampus, damage to acetylcholine-containing neurons is associated with the memory loss seen in people with Alzheimer’s disease. The neurotransmitter is also involved in sleep and extrapyramidal motor regulation.
Acetylcholine-sensitive receptors are called cholinergic receptors. In this context, the -ergic suffix means “activated by,” so the term cholinergic just means “activated by choline.” (Choline is one of the chemicals that acetylcholine is made out of, which is why it is in the name.) There are two types of cholinergic receptors: muscarinic and nicotinic. Both are named after plant substances (muscarine and nicotine) that produce non-overlapping effects induced by acetylcholine. The nicotinic receptor subtypes are all ionotropic receptors, while the muscarinic receptor subtypes are largely metabotropic receptors.
4.2.2. Norepinephrine, Epinephrine, and Dopamine
In this subsection, we will cover catecholamines, which are organic compounds that consist of a catechol (a benzene ring with two hydroxyl side groups) connected by two carbons to a single amine (NH) group. You don’t need to memorize the molecular structure of each neurotransmitter for this class, but examining their chemical structures will reveal similarities between each of the neurotransmitters in this class.
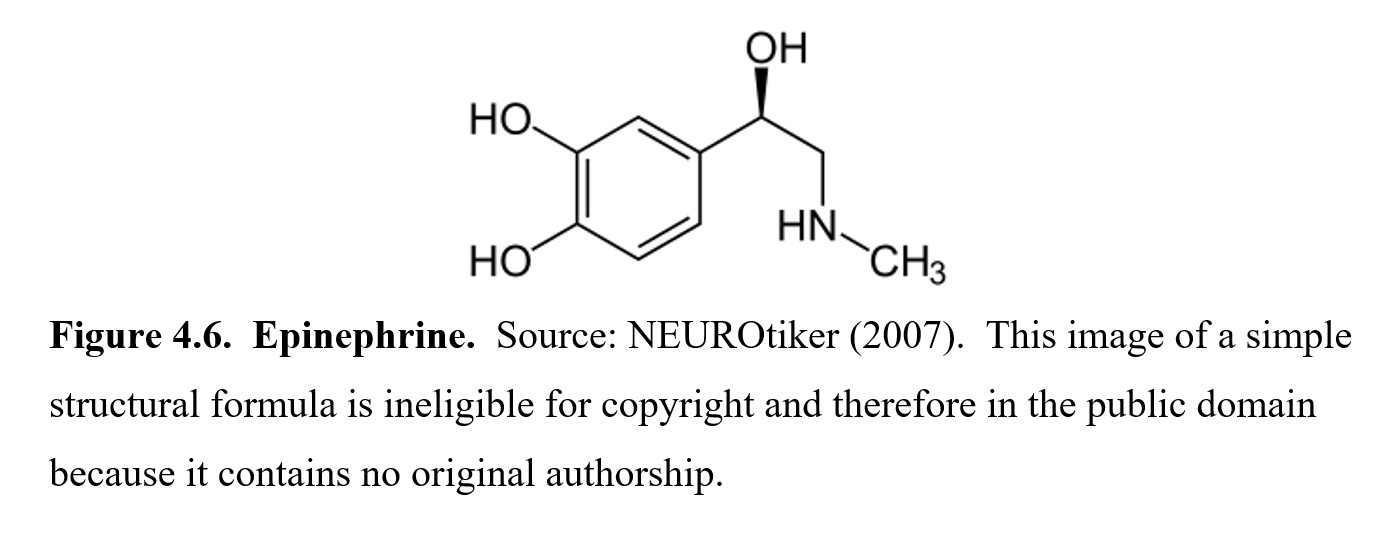
The first catecholamine we will discuss is epinephrine. In the UK and Europe, epinephrine is called adrenaline, which probably gives you a sense of what epinephrine does. We often talk about getting an adrenaline rush in fight-or-flight situations, so as you might expect, epinephrine is involved in the sympathetic nervous system. Epinephrine can dilate the pupils and increase blood flow from the heart to the muscles. It is a hormone that is produced by the adrenal glands and activates adrenergic receptors, of which there are two main types called alpha (α) and beta (β) adrenergic receptors. You might also see adrenergic receptors called adrenoreceptors, which is just a shorter name for the same thing.
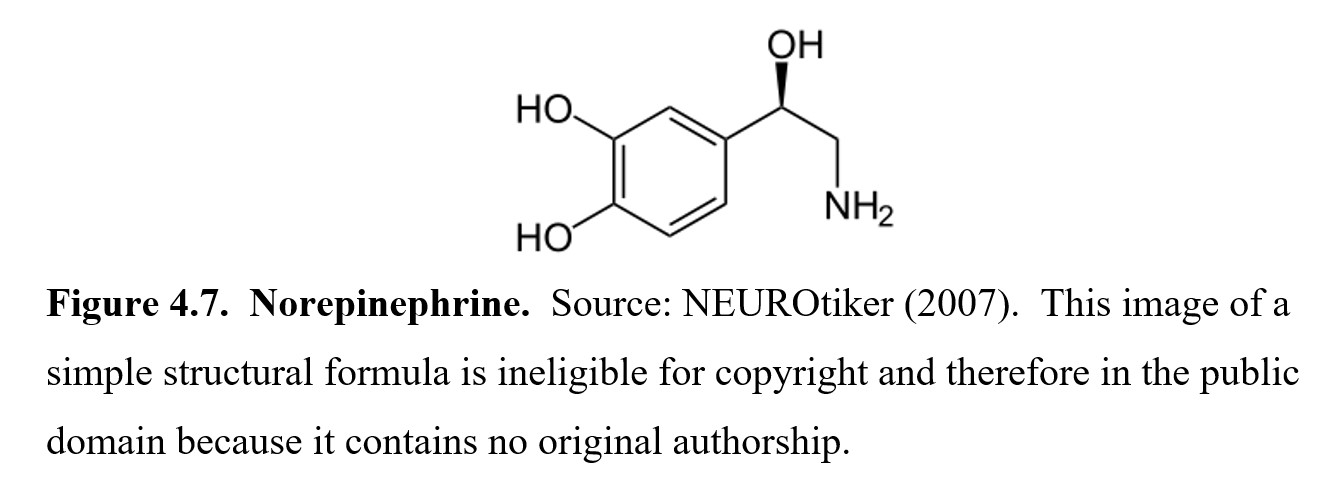
A very similar chemical is called norepinephrine, which is also called noradrenaline internationally. The nor- prefix comes from the fact that in this molecule, the CH3 group is missing from the nitrogen. Hence, nor- (from Nitrogen ohne Radikal in German for “nitrogen without the radical”) Is added to epinephrine to indicate this difference. Norepinephrine is the working neurotransmitter of the sympathetic nervous system, and, similar to epinephrine, it activates alpha- and beta-adrenergic receptors and is involved in the sympathetic “fight-or-flight” response.
Unlike epinephrine, which is released solely as a hormone, norepinephrine is released as both an adrenal hormone and a typical neurotransmitter. In the CNS, it increases arousal and attention and plays a role in arousal and attention as well as in mood and appetite regulation. A deficiency in norepinephrine can be a component of certain types of depression, which we will cover in detail when we discuss antidepressants. A deficit in norepinephrine neurotransmission may also be involved in certain types of attention deficit hyperactivity disorder.
What is the difference between a hormone and a neurotransmitter?
We mentioned that epinephrine is a hormone secreted by the adrenal gland, but what exactly is a hormone? Hormones are produced in organs called glands and are secreted into the bloodstream. Compare this to most neurotransmitters, which are produced inside the axon terminal of neurons and released into the synaptic cleft. The big difference is range: hormones are circulated throughout the body and can reach distant target cells, while neurotransmitters are limited to the 20- to 40-nm gap in the synapse.
Although there is a clear line between hormones and neurotransmitters in terms of function, some chemicals can behave as neurotransmitters in some cases and as hormones in others. If you are interested in learning more—it is not required for this class—a good place to start is this brief writeup by Alpana and Murari Chaudhuri:
Hormones and Neurotransmitters: The Differences and Curious Similarities
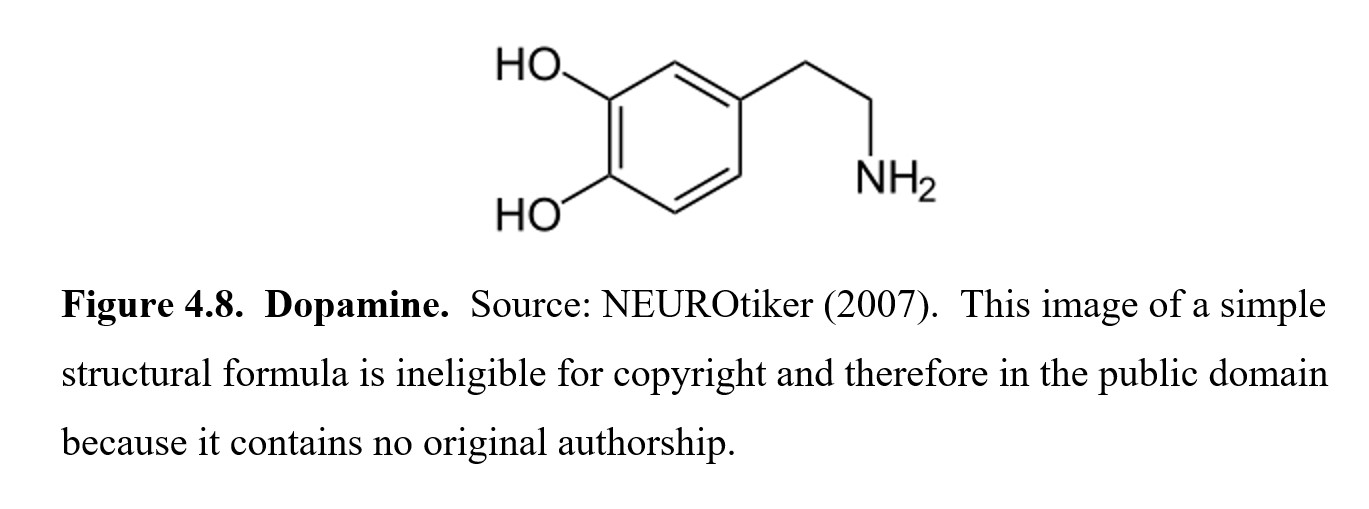
The third and final catecholamine is dopamine. Dopamine is a very important component of drug dependence, as one main role of dopamine in the CNS is reward and reinforcement. The addictive properties of many drugs come from how the drugs stimulate dopamine release and create long-term changes in dopamine-related pathways in the brain. Dopamine is also involved in motor control. Two well-known neurological disorders, Parkinson’s disease, and Huntington’s disease are caused by a deficiency (Parkinson’s) or excess (Huntington’s) in dopamine activity in the basal ganglia. Dopamine also plays an important role in nausea and vomiting and regulation of the pituitary hormone prolactin. The psychiatric disorder schizophrenia is also thought to be caused by an excess of limbic dopamine activity
Dopamine activates dopaminergic receptors, of which there are at least five different subtypes (D1, D2, D3 D4, D5). Dopamine receptors are metabotropic receptors that are found predominantly in the CNS.
4.2.3. Serotonin and Histamine
The three catecholamines mentioned above are actually a subset of a larger group of neurotransmitters called monoamines because they have a single amino group connected to an aromatic ring by a two-carbon chain. It is less important that you can explain the molecular structure of monoamines. Instead, you should be able to name the five neurotransmitters that are classified as monoamines—the three catecholamines listed above and the two covered below.
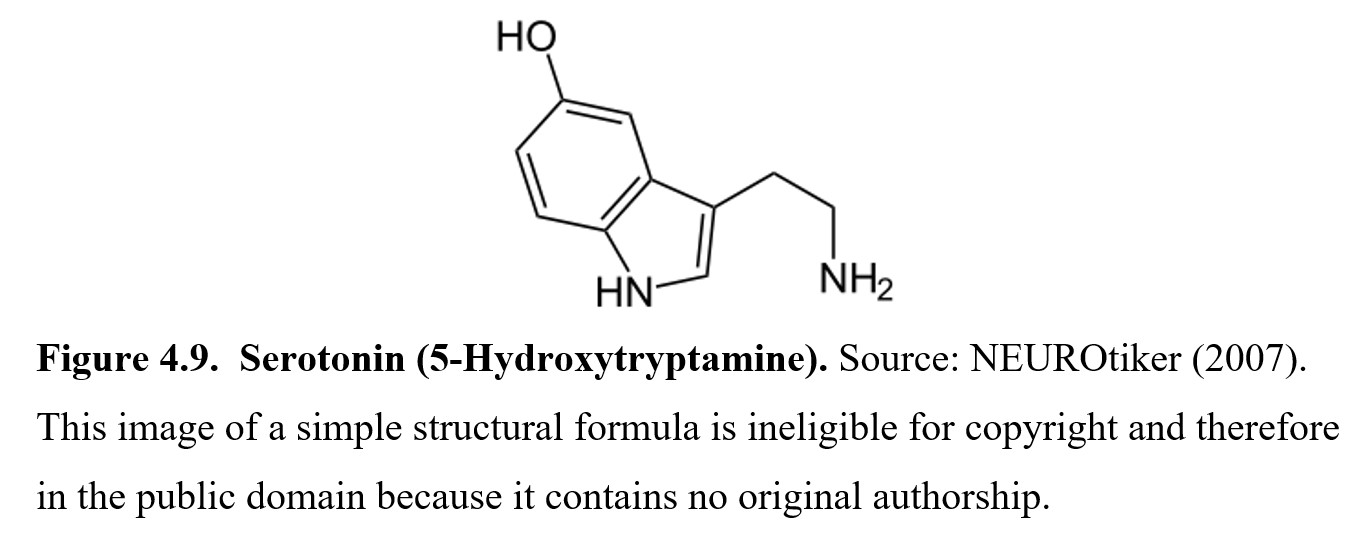
The fourth monoamine is serotonin, which is classified as an indoleamine because of the two-ring indole nucleus connected by a two-carbon chain to the amine group. The full chemical name for serotonin is 5-hydroxytryptamine. You will often see serotonin referred to as 5HT in the scientific literature, so it is worth remembering.
In the PNS, serotonin plays a large role in digestion. Ninety percent of the serotonin in the body is produced in the digestive tract, where it regulates intestinal movement, gastric acid secretion, and mucus production. In the CNS, serotonin is linked to numerous functions, including sleep, anxiety, mood, appetite, nausea, and social and sexual behavior. Similar to norepinephrine, one type of depression is classified as being due to a serotonin deficiency. 5HT also plays a major role in the genesis of anxiety.
Serotonin receptors are called 5HT receptors. They are the most abundant in the human body and contain the most subtypes out of all receptors. They are grouped into 7 main types, labeled 5HT1 to 5HT7, and each of those can be distinguished further into subtypes like 5HT1A, 5HT1B, and 5HT2C. Some 5HT receptor subtypes are metabotropic while others are ionotropic. Moreover, some 5HT receptor subtypes are excitatory and some are inhibitory.
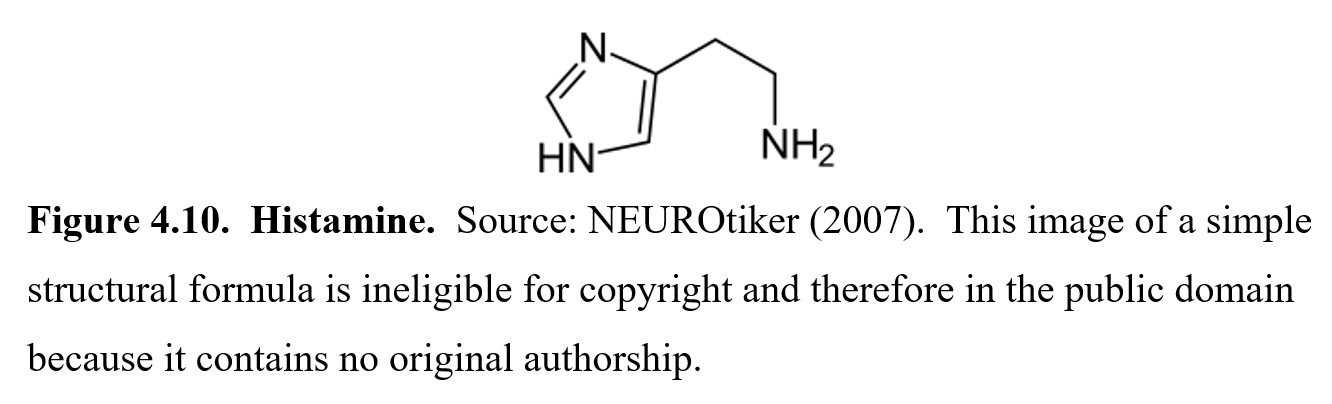
The last monoamine we will cover is histamine (there are other monoamines, but we will focus on these five for this class. In the PNS, histamine is a part of the inflammatory response, which helps the immune system fight pathogens. This response is what causes itching, sneezing, and a runny nose when you have a cold. In the CNS, histamine is involved in a variety of effects, the most important of which is promoting wakefulness. This is why antihistamine medications are well-known for causing drowsiness and sedation. Histamine acts at four main H receptors (H1 to H4) all of which are metabotropic.
4.2.4. Glutamate, GABA, and Glycine
The next group of neurotransmitters is the amino acid transmitters. You may recall learning that amino acids are the building blocks of proteins. There are a few amino acids that also act as neurotransmitters. In this subsection, we will look at the three most important ones.
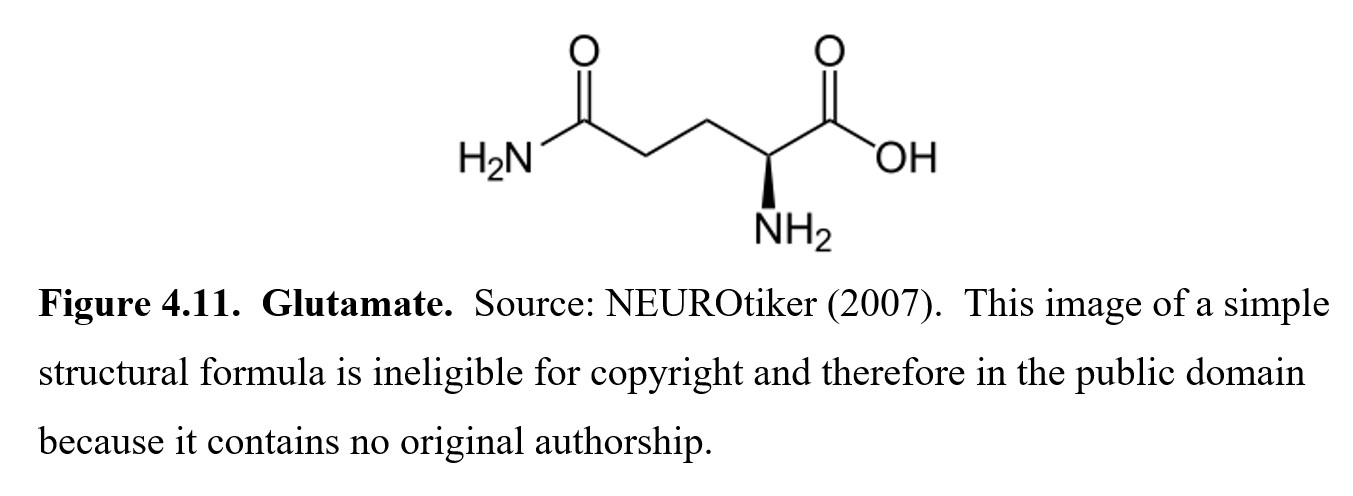
The first is glutamate, which is the main excitatory neurotransmitter in the CNS. It is involved in various cognitive functions like learning and memory. It is also implicated in neurological conditions such as Alzheimer’s disease, Parkinson’s disease, and epilepsy through a process called excitotoxicity, where overstimulation of glutamate receptors can result in cell degradation and eventually cell death.
The three main glutamate-sensitive ionotropic receptors are named for the agonist that activate them: NMDA (N -methyl-D-aspartate); AMPA (α-amino-3-hydroxy-5-methyl-4-isoxazole-propionic acid); and kainate receptors. The first of these is of particular interest to this class since NMDA receptors are the target sites for certain psychoactive substances like PCP and ketamine as well as glutamate-induced excitotoxicity. Other species of glutamate-sensitive receptors are metabotropic in nature. These are simply called metabotropic glutamate receptors (mGluR) and have several subtypes.
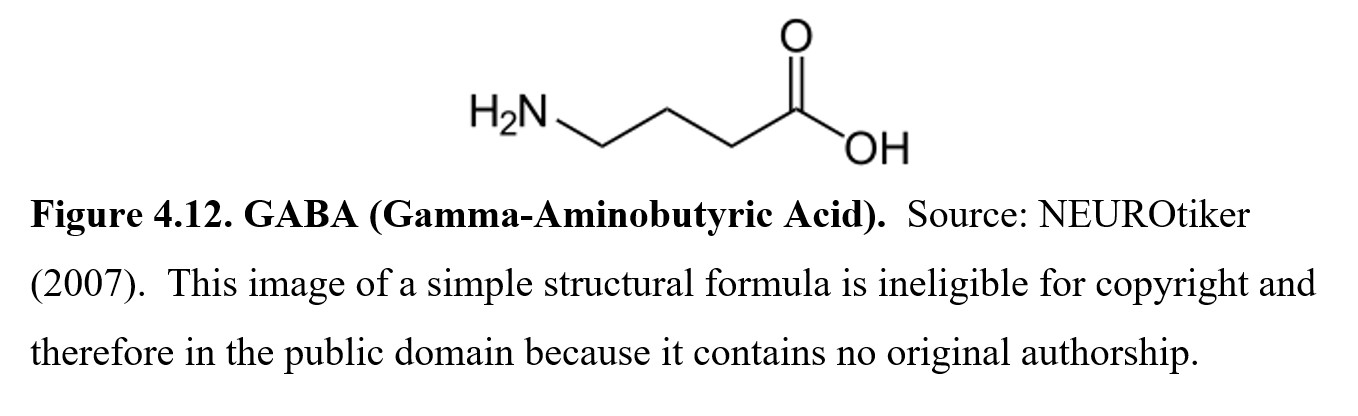
The next amino acid neurotransmitter is called γ-aminobutyric acid or gamma-aminobutyric acid (GABA). It is the principal inhibitory neurotransmitter in the brain, meaning that glutamate and GABA have opposing effects. GABA acts at two receptor subtypes, called GABAA and GABAB, to dampen overactive neurons. Many sedatives and tranquilizers achieve their effects by enhancing GABA transmission in some way. Because it is involved in a wide variety of CNS depressants, including alcohol, we will cover GABA and its receptors in more detail in a later chapter. GABA may also have actions at other types of receptors under investigation.
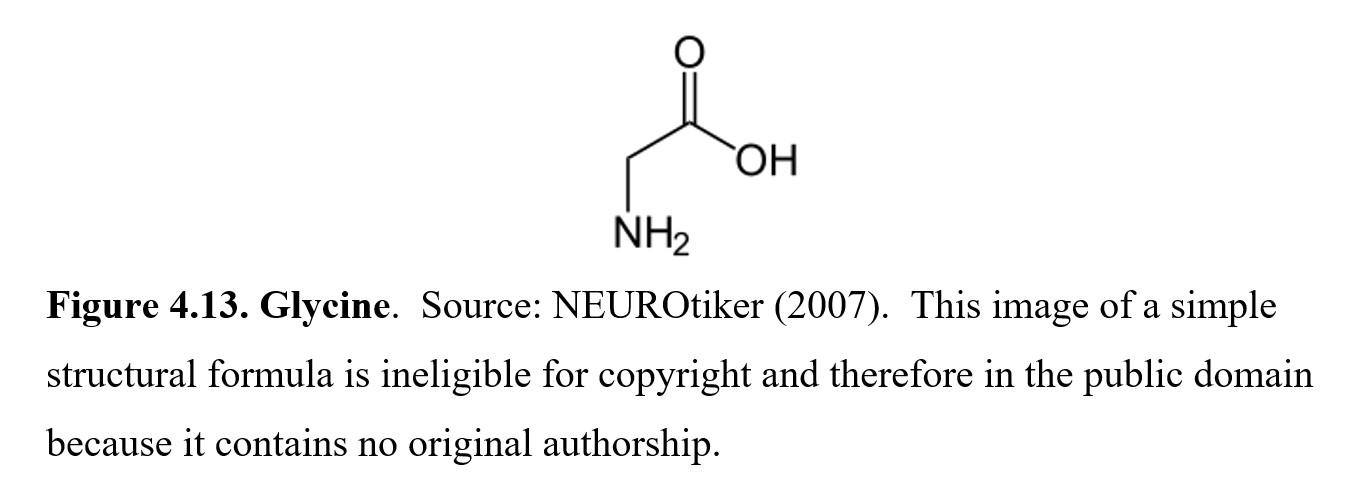
Finally, whereas GABA is the main inhibitory transmitter of the brain, glycine is the main inhibitory transmitter in the spinal cord, although it is also present in the brainstem and retina. The glycine receptor (GlyR) is a ligand-gated chloride ion channel. Aside from its role as an inhibitory transmitter like GABA, it is also involved in processing motor and sensory information.
4.2.5. Endorphins and Substance P
So far, all the neurotransmitters we’ve covered are considered small molecule transmitters, since their chemical structures are centered on small molecules such as choline or amino acids such as tyrosine, tryptophan, or histidine. Some neurotransmitters can be much larger, however. Such transmitters are called peptides or neuropeptides; peptide means that they are comprised of a chain of amino acids, similar to proteins (although much less complex). We will cover two types of relevant neuropeptides: endorphins and neurokinins (Substance P).
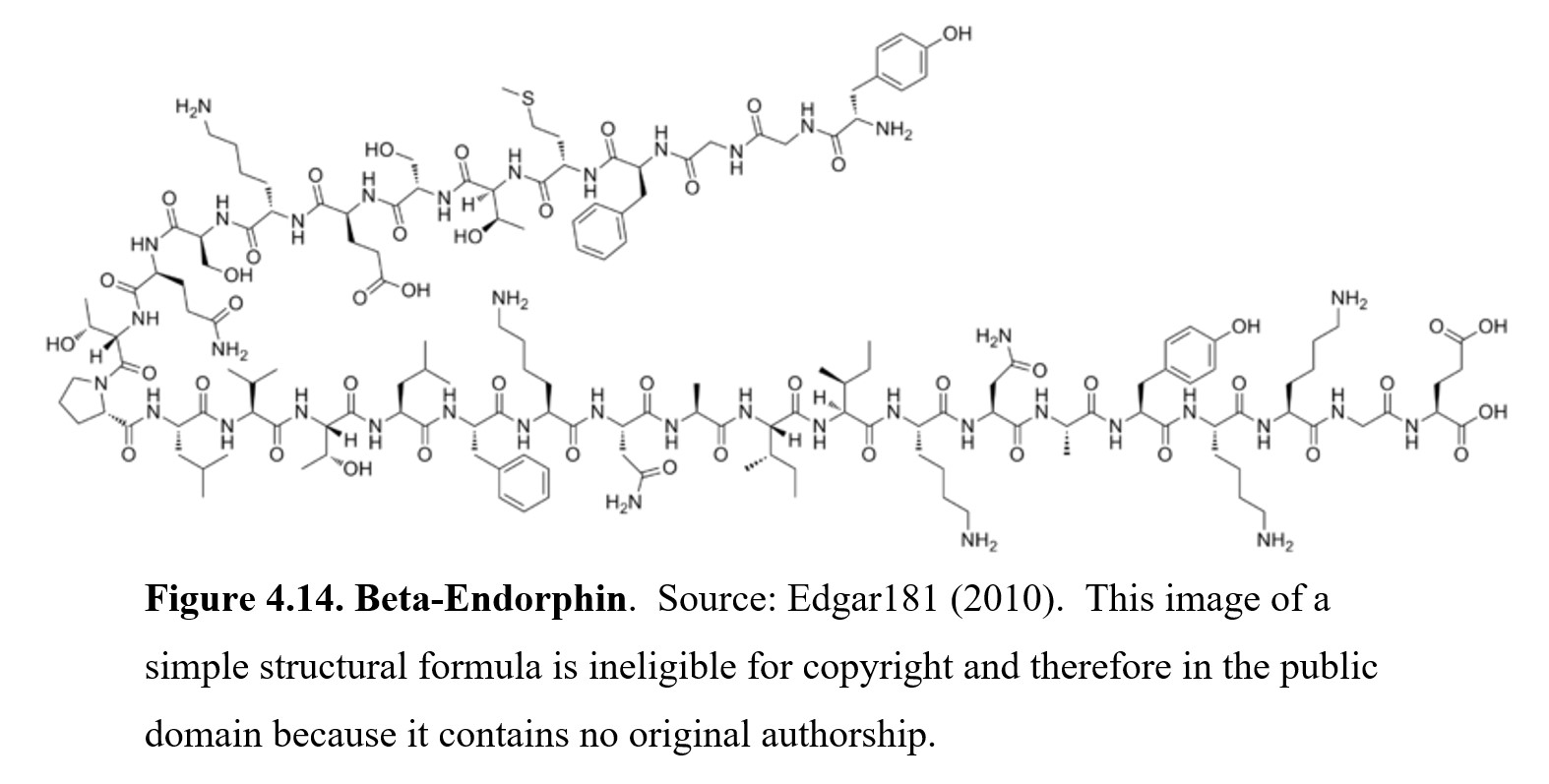
The first type of neuropeptide we will look at are the endorphins, The name endorphin comes from a contraction of endogenous morphine since they occur naturally in the body. Above you can see the structure of beta-endorphin. It is humungous compared to all of the transmitters we have looked at so far. Beta-endorphin consists of 31 amino acids. Endorphins act at opioid receptors, of which there are also types: mu (μ), kappa (κ), and delta (δ). Beta-endorphin acts primarily at mu-opioid receptors similar to morphine.
Endogenous opioid peptides are noted for producing an analgesic (pain-blocking) effect. This is because the activation of opioid receptors inhibits the release of Substance P, which we will discuss next. In the PNS, endorphins (mostly beta-endorphin) are secreted by the pituitary gland and act as hormones to relieve stress and pain. In the CNS, endorphins also trigger feelings of pleasure by inhibiting GABA, which in turn increases dopamine activity. The euphoric sensations that occur from listening to music, eating something delicious, having sex, or engaging in vigorous aerobic exercise (known as a “runner’s high”) are all the result of endorphin activity. You may have heard of the term “runner’s high,” which refers to the relaxed state of bliss following an intense exercise.
Beta-endorphin and two additional endogenous opioid peptides, enkephalins and dynorphins, all have the same N-terminal amino acid sequences of Tyr-Gly-Gly-Phe. Enkephalins are pentapeptides (five amino acids) that possess affinity and efficacy for opioid receptors. Two enkephalins have been widely studied, methionine-enkephalin and leucine-enkephalin. Like beta-endorphin, they are analgesic and are released in times of stress. Enkephalins can bind to mu- and delta-opioid receptors with moderate selectivity for the delta-opioid receptor.
Dynorphins constitute the third major type of endogenous opioid peptides and are derived from a larger precursor protein prodynorphin. There are no less than six different dynorphins of which are fragments of a 32-amino acid precursor. The most widely studied dynorphin is dynorphin A1-8, which can act upon mu-, kappa-, and delta-opioid receptors with its greatest affinity for the kappa-opioid subtype. Dynorphin has been identified as the endogenous kappa-opioid ligand with roles in pain and stress response, learning, and memory. There is interest in the development of drugs that act upon kappa-opioid receptors because they produce pain relief without minimal adverse side effects such as respiratory depression, constipation, and addiction.
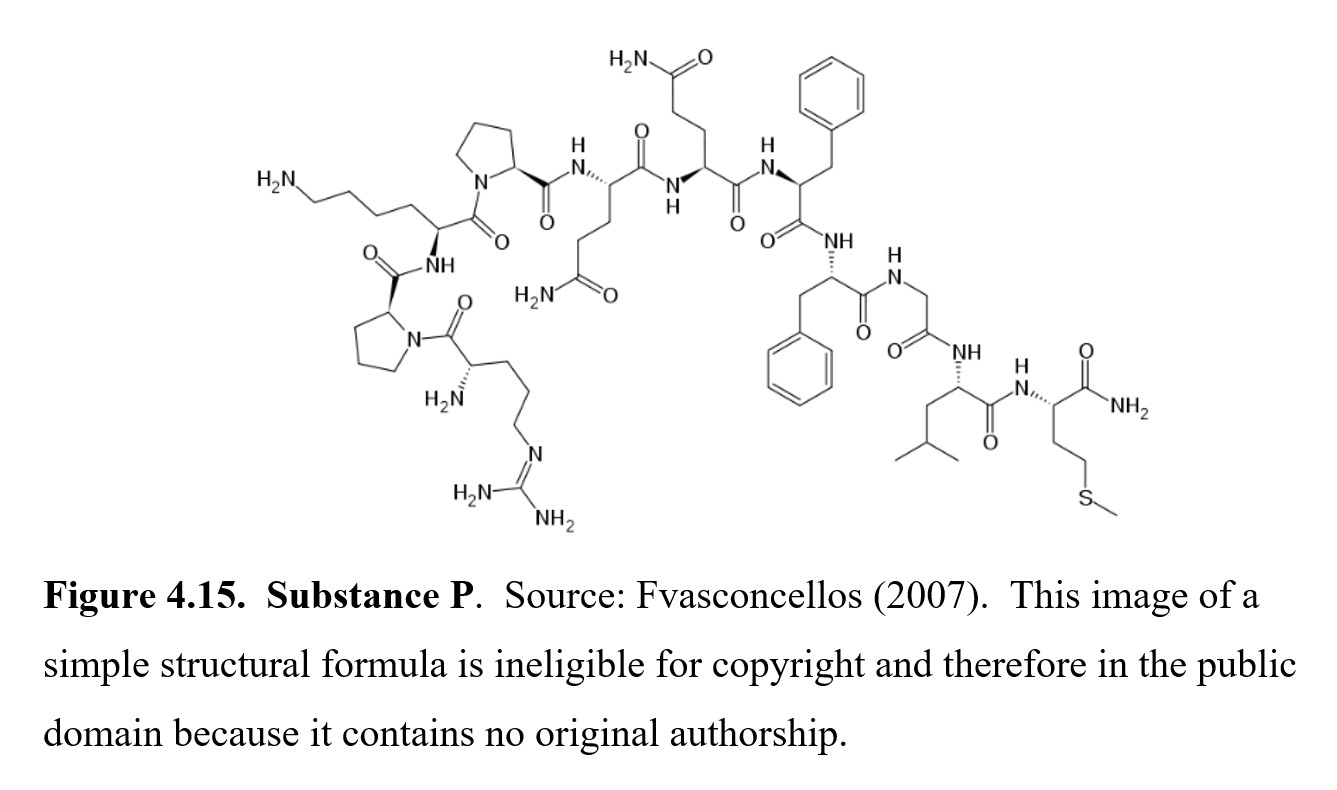
Neurokinins (also known as tachykinins) are neuropeptides involved in nociceptive processing and other functions. Substance P was the first neuropeptide discovered and purified in powder form (hence, the P in its name). It is an 11-amino acid peptidethat is released from the ends of sensory nerves and activates neurokinin-1 (NK1) receptors to initiate pain signals that travel up the ascending nociceptive pathway to the brain. Curiously, there are available drugs that can block Substance P-sensitive receptors, but they do not produce clinical analgesia in human subjects.
4.2.6. Nitric Oxide
Aside from small molecule neurotransmitters and peptides, there is a third and newer type of transmitter called gaseous signaling molecules or gasotransmitters. These are gas molecules that can freely permeate cell membranes. Instead of binding to receptors on the surface of a cell like all other neurotransmitters discussed previously, these molecules simply diffuse across the membrane on their own and alter cell physiology directly inside the cell. Because they can permeate cell membranes, they cannot be stored in vesicles like other neurotransmitters. Instead, they are produced on demand. Gaseous signaling molecules are relatively recent discoveries in neurotransmission. Much is currently unknown about them, so we will only look at the one that has been studied the most.
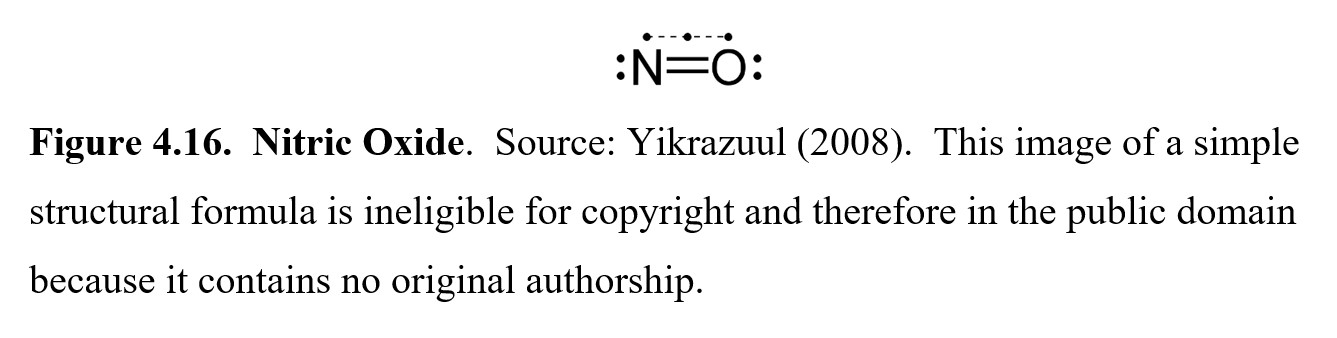
Nitric oxide (abbreviated NO) is a simple molecule comprised of a single nitrogen atom coupled to a single oxygen atom. NO is a free radical, meaning it has an unpaired electron. The “receptors” that it binds to are enzymes inside the cell, of which the most researched is soluble guanylate cyclase (SGC), which converts guanosine triphosphate (GTP) to cyclic guanosine monophosphate (cyclic GMP), a second messenger within cells. When NO binds to the enzyme, it triggers a signal cascade that leads to the relaxation of smooth muscle among other effects. Because of this, a common use of nitric oxide is to dilate blood vessels and increase blood supply in cases of acute respiratory failure and pulmonary hypertension. In 1998, the Nobel Prize in Physiology or Medicine was awarded to three scientists for their discoveries leading to the recognition of NO as a bioregulator in the cardiovascular system.
Nitric oxide also plays a role in the immune system, as it is toxic to cellular organisms and can be secreted in response to pathogens. Studies have suggested that it can inhibit the replication of certain viruses such as severe acute respiratory syndrome (SARS) which has led to nitric oxide being proposed as a therapeutic option for treatment of COVID-19 by mitigating severe acute respiratory distress syndrome.
4.2.7. Transmitters and Receptors Review
Phew, that was a lot to cover. If you got a little lost at times, it is okay—there were lots of new terms being thrown around. To learn this material and organize it in your head, you will have to start by memorizing the associations between the neurotransmitters, their receptors, and their functions. Creating flashcards is a great way to practice and form those connections. Here is a humorous way of summarizing the more important neurochemical transmitters
The Neurotransmitter Song [5:10]
To help you, Table 4.1 below summarizes all the important information from this section.
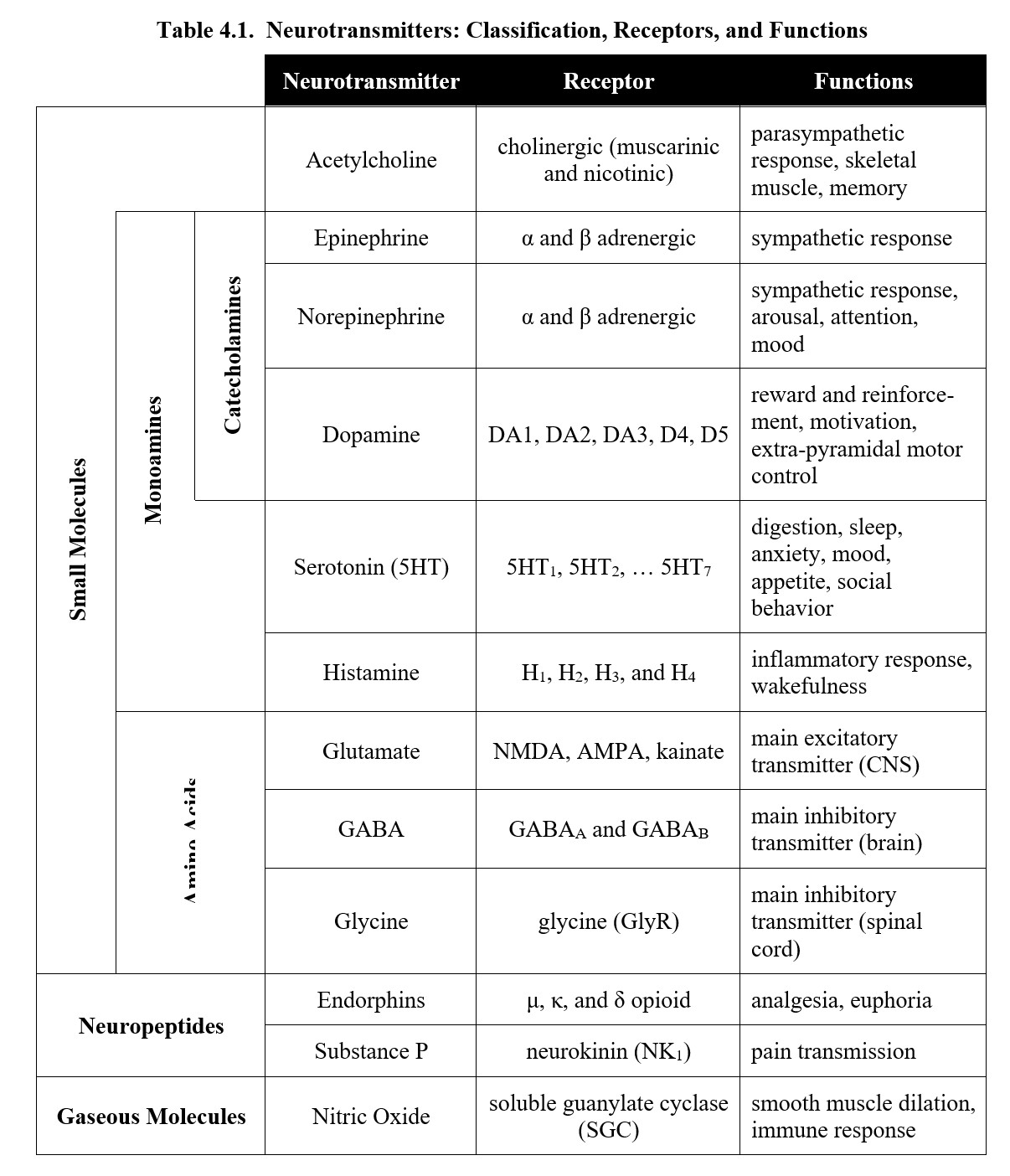
Chapter Summary and Review
In this chapter, we took a deep dive into neurotransmitters, starting with how they were discovered and how they worked in the synapse. We explored the different types of receptors that they could activate and how other substances could interact with those receptors. Finally, we provided an overview of a handful of important neurotransmitter/receptor pairs, including how they are classified and what functions they are involved in.
Good work on making it to the end of the neuroscience chapters. This chapter contained many new terms that will be used throughout this course. Thus, testing yourself with flashcards is highly recommended. As in previous chapters, you can check your understanding with the practice questions below. Remember, they are not comprehensive and are only meant as a starting point.
Chapter 4 Practice Questions
Answer the following questions:
- Who discovered the concept of neurotransmission?
- Name three different ways that neurotransmitters can be removed from the synaptic cleft.
- Describe the differences between ionotropic and metabotropic receptors.
- What is the difference between a ligand that has a high affinity for a receptor and one that has a high efficacy?
- What are the two types of receptors activated by acetylcholine?
- Name the five monoamines covered in this chapter. Which ones are the catecholamines?
- What neurotransmitter is highly associated with reward and motivation?
- What is another name for serotonin?
- Name the three amino acid neurotransmitters and where each is found in the nervous system.
- Which two neurotransmitters are considered the main excitatory and inhibitory transmitters in the brain?
- What type of receptors are activated by endogenous opioid peptides?
- What is the role of Substance in the perception of pain?
- What are endogenous opioid peptides?
- Discuss the unique features of gasotransmitters, using nitric oxide as the prime example.
- Describe the differences between small molecules, peptides, and gaseous signaling molecules.
- How can drugs influence physiological function by affecting neurotransmission?
2nd edition